Multi-use of offshore wind farms with low-trophic aquaculture can ... - Nature.com
Abstract
Multi-use of offshore wind farms with low-trophic aquaculture could provide sustainable energy, nutritious seafood, and restorative ecosystem services through nutrients and carbon capture and utilization. In a transition zone between marine and brackish seas, our model predicted that allocating 10% of projected wind farm areas to blue mussel and sugar kelp aquaculture in the North Sea - Baltic Sea transition zone could yield 18 t-fresh weight ha−1 yr−1. Total carbon captured and harvested from seaweed biomass and mussel shells would equal 40% of the carbon dioxide emissions from the Danish agricultural sector. Furthermore, global aquaculture production is projected to increase by 132% compared to current production. With technological and regulatory challenges still to be addressed, these findings demonstrate a vast potential of multi-use in offshore areas, which can generate blue biomass with fewer user conflicts, while mitigating eutrophication and climate change, thereby supporting multiple global sustainable development goals.
Introduction
The coastal zone is heavily impacted by human activities and the demand for space is continuously increasing1. European coastal seas, in particular, host a wide range of different competitive uses, such as shipping, oil and gas exploitation, renewable energy production farms, mining, disposal, recreational activities, aquaculture, fishing, and conservation2,3. This diversity and intensity of exploitation of the coastal zone substantially increases the stress on marine ecosystems, leads to spatial conflicts, and hinders the expansion of many of these uses. Sustainable development of the blue economy requires area-efficient use of marine space and innovative solutions demonstrating net emission capture thereby contributing to mitigating eutrophication and climate change4. One potential solution is the offshore integration and relocation of human activities where more generous expansion opportunities exist because of reduced conflicts with other interests e.g., exploitation or recreational5,6. Concentrating uses in designated offshore marine areas could provide infrastructural, economic, and environmental benefits through a multi-use approach7, while allowing other areas to remain less impacted or be designated as strictly protected areas (MPAs). Co-locating offshore wind farms (OWF) with low-trophic aquaculture (LTA) has been suggested as an efficient multi-use strategy providing emission-free energy, nutritious seafood, and restorative ecosystem services (ESS) through capture and utilization of emission (carbon dioxide (CO2) and nutrients) (Fig. 1)5,8,9,10,11. ESS are defined as 'the contributions that ecosystems make to human well-being, and are distinct from the goods and benefits that people subsequently derive from them' sensu12.
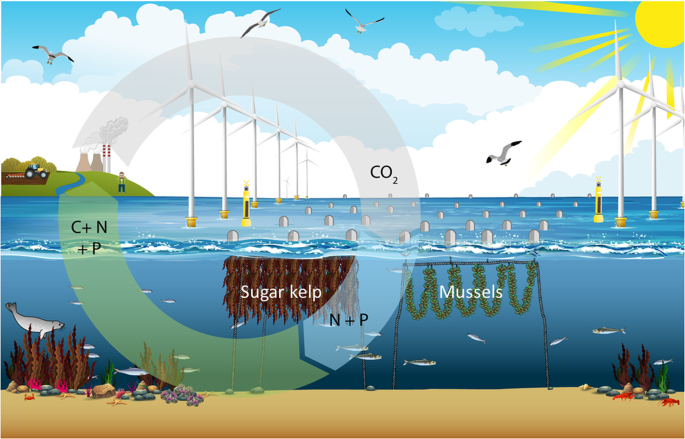
Multi-use in offshore wind farms with farming of blue mussels and sugar kelp can deliver emission free energy, nutritious seafood, and positive ecosystem services through emission (CO2 and nutrients) capture and utilization.
LTA delivers important ESS such as the provisioning of food and feed with fewer resources required and a low carbon footprint compared to terrestrial protein production9,13,14. In addition, the extractive species of LTA take up nutrients and store carbon from the water column11. CO2 and dissolved nutrients are built into seaweed biomass through photosynthesis, uptake, and growth. CO2 is built into mussel shells by bio-calcification, whereas organic carbon (C) and nutrients are incorporated into mussel tissue through filtration of suspended particulate organic matter4,15,16,17. Hence, harvesting of bivalves and seaweeds removes nitrogen (N) and phosphorous (P) from the sea, leading to improve water quality, especially in eutrophic areas18,19. Additionally, seaweed photosynthesis contributes to increased pH and oxygen, effectively mitigating local ocean acidification20 and de-oxygenation21. LTA can further contribute to climate change mitigation by promoting a shift in people's diet from terrestrial animal-based proteins to seafood with a lower carbon footprint14,22. As harvesting offers the potential for efficient recirculation of nutrients and carbon from sea to land, LTA also makes a positive contribution to circular bioeconomy23. Coastal communities, however, show concerns with respect to the visual disruption of the waterscape, competition for space, generation of waste, negative influence on tourism, lack of market, economic viability, and inappropriate regulations24,25. As a result, the expansion of LTA, e.g., bivalves, has been hindered in Europe over the last two decades26. The European Union has accordingly funded several projects under the umbrella of Blue Growth Strategies, Horizon 2020, and Horizon Europe, where the use of the open ocean for LTAs has been promoted with the inclusion of economic and social aspects.
Worldwide, the number of marine wind farms producing renewable energy is steadily increasing and considered a sustainable alternative to the use of fossil energy3,5,27,28. However, opposition exists against more wind farms along the recreationally valuable coastline, because of esthetics and interference with the landscape image. Locating wind farms further offshore would solve this issue and at the same time ensure better wind conditions for increased energy production.
Several projects in northern Europe have modeled and tested if offshore LTA could be spatially combined with OWF in the areas that are otherwise restricted due to the safety of OWF operations and excessive infrastructure costs5,29. These feasibility studies demonstrated that the multi-use of OWF combined with aquaculture of blue mussels (Mytilus spp.) and sugar kelp (Saccharina latissima) was promising in the saline North Sea30,31. In the brackish Baltic Sea, however, the growth of aquaculture species is hampered due to low salinity4,32,33,34. In eutrophic, low saline areas, LTA can be used as a tool to remove nutrients (referred to as mitigation culture) rather than production for direct consumption23,34,35. Mussel mitigation culture involves a higher mussel density and the mussels are typically smaller and more variable in size compared to those commercially produced for human consumption36. The upscaling of OWF-LTA to commercial scale depends on the technological readiness, production potential, environmental conditions and considerations, economic viability, permits and regulations, and operational difficulties caused by the interaction between the two or more co-uses, calling for joint efforts in risk governance5,30,31. The development of OWF-LTA is important to meet the global sustainable development goals (SDGs) about supporting marine life (#14), food security (#2), affordable and clean energy (#7), climate action (#13), responsible consumption and production (#12), and partnership for the goals (#17)18,37,38.
The aim of the present study is to explore and model the OWF-LTA concept on both regional and global scales, as a mean to contribute to multiple global SDGs. The potential is exemplified using data from the North Sea-Baltic Sea transition zone, where the construction of new OWFs of substantial scale is planned by the National Authorities to be realized before 2030 (Supplementary Table 1, Fig. 2). Specifically, the study predicts (i) future OWF area development with potential for multi-use with LTA, (ii) potential biomass yield of blue mussel and sugar kelp available as a bio-resource for food, feed, and materials, and (iii) potential emission capture and utilization of C, N, and P by LTA for the study area and upscaled in a global context for similar LTA species in seven different regions using spatial models.
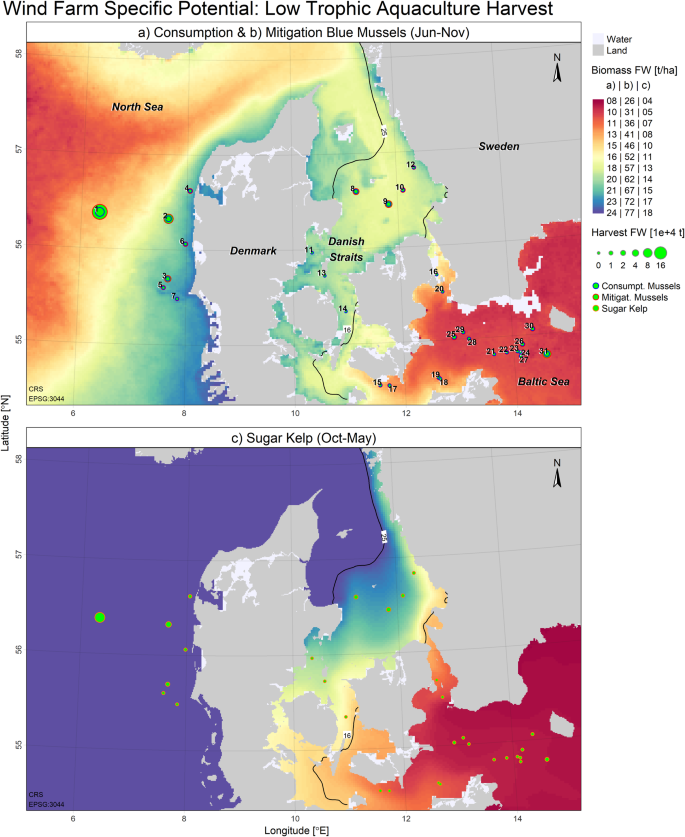
Maps showing the harvest potential (104 t-FW yr−1) of (a) commercial mussel culture for consumption, (b) mussel mitigation culture, and (c) sugar kelp for each OWF (green circles) indicated with an ID number sorted after decreasing average salinity (#1 to #31). The background colors show the area-specific harvest potential (t-FW ha−1). The solid lines indicate the salinity borders between the three areas: the North Sea (west, >25 psu), the Danish Straits (middle, 16–25 psu), and the Baltic Sea (east, 8–16 psu). Above 25 psu, sugar kelp is assumed to grow optimally with respect to salinity, but other growth determining factors are not included here.
Results
OWF development
Information regarding OWF development was obtained from the 4C global offshore database and used to create three projections (realistic, pessimistic, and optimistic) based on 12 project status categories (see method section) for marine waters (Fig. 3, Supplementary Tables 2 and 3). For the North Sea–Baltic Sea transition zone, the realistic projected OWF size is estimated to increase three-fold reaching 325 K-ha distributed over 169 K-ha in the North Sea, 36 K-ha in the Danish Straits, and 120 K-ha in the Baltic Sea (Table 1). Globally, Northern Europe (including the study area) showed the highest OWF development potential. In the realistic projection, the region is expected to experience a nine-fold increase from 538 K-ha in 2023 to 4843 K-ha by 2030 (Fig. 3). The pessimistic projection was 1186 K-ha, while the optimistic projection was 20,809 K-ha for Northern Europe. Globally, the OWF areas are expected to increase 13, 2, and 49 times in realistic, pessimistic, and optimistic projections, respectively, compared to the current situation (Fig. 3). Asia also showed large OWF expansion potential in all scenarios but with lower percentages in the realistic and optimistic projections. The regions Africa, Australia-New Zealand (NZ), North- and South America, and southern Europe have currently no or few OWF, but all these regions are expected to invest in OWF in the realistic- and optimistic projections (Fig. 3).
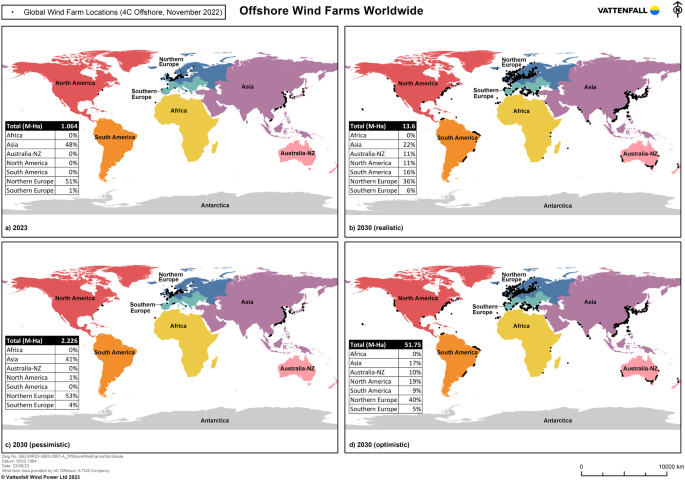
Global locations of the OWF for (a) the 2023 situation, (b) the realistic 2030 projection, (c) the pessimistic projection, and (d) the optimistic projection. (see methods for definition of the scenarios). The tables show total OWF area (M-ha) and percentage distribution across the regions.
Harvest potential in the study area
Salinity is an important factor for LTA harvest yield due to the hampering of species growth at lower salinities in the study area. Spatial models of harvest potential were applied for blue mussels and sugar kelp. The blue mussel harvest model was a function of temperature, chlorophyll a (Chl a), and salinity. Model calculations of the critical flow velocity ratio (0.65 to 21) showed that there was sufficient food supply to avoid food limitation for the mussels at all sites. Mussel farms at salinity >16 psu were configured as commercial culture (human consumption), whereas the mussel farms at lower salinity were configured as nutrient mitigation culture. The total production potential of commercial mussel farms in the more saline North Sea and Danish Straits (>16 psu) showed an average area-specific harvest potential of 16–18 t-fresh weight (FW) ha−1 y−1 (Table 1, Fig. 2). In the brackish Baltic Sea (8–16 psu), mussel harvest potential was considerably higher with 33 t-FW ha−1 y−1 because of higher densities of small mussels (not suited for human consumption) with lower growth rates designed to remove as much nutrients as possible per farm area.
The sugar kelp harvest model was a function of salinity only, since salinity is the dominating environmental factor determining the yield potential of kelp in brackish waters. It is assumed that sugar kelp is harvested before potentially high summer temperatures limit productivity (see method section). The sugar kelp model predicted a 3–4 times higher harvest potential of 16–18 t-FW ha−1 y−1 in the more saline North Sea and Danish Straits compared to the brackish Baltic Sea (Table 1, Fig. 2). The model assumed that nutrient limitation for sugar kelp aquaculture within OWF regions are unlikely33. Our deployment of sugar kelp farms, covering just 10% of the wind farm area, and the strong water exchange typical of offshore areas, reduce this possibility. Overall, potential LTA harvest was 17 t-FW ha−1 y−1 and 19 t-FW ha−1 y−1 at salinities >16 and <16, respectively, assuming a 50/50 areal distribution of mussels and sugar kelp.
According to the experience with wind farm operators and other multi-use projects, not all the area between wind turbines can be used for LTA. This is because as accessible routes to the wind turbine must be maintained and safety zones around the wind farm area, and within the windfarm around cables, and the wind turbines. Additionally, this can be further combined with other multi-use scenarios (e.g., ecotourism, passive fishing, nature conservation) that also require space. Based on this, we have made a conservative calculation of the possible OWF-LTA areas, which is derived from the long-term results of other multi-use projects at both the national level (Germany) and international level (EU)39,40,41. When allocating 10% of OWF to LTA (i.e., a conservative regional scenario), the total LTA harvest potential reached 578 Kt-FW y−1 with the yields being higher for blue mussels than sugar kelp (365 Kt-FW y−1 mussels and 213 Kt-FW y−1 seaweed; Fig. 2). Total LTA harvest potential was highest in the North Sea with 290 Kt-FW y−1, followed by 226 Kt-FW y−1 in Baltic Sea, and lowest with 62 Kt-FW y−1 in the Danish Straits due to the smaller allocated area (Table 1, Fig. 4).
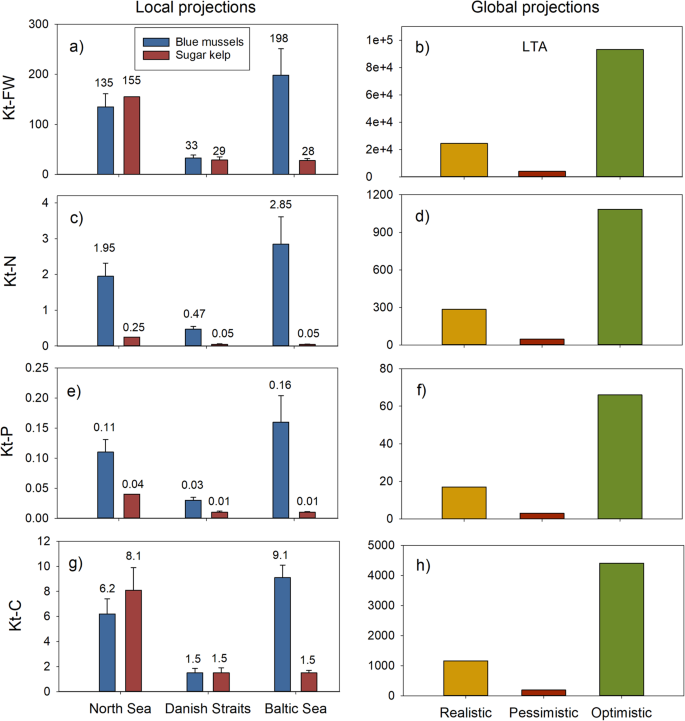
Potential total FW-harvest (t-FW yr−1) (a–b) and related removal of N (c–d), P (e–f) and C (g–h) by blue mussels and sugar kelp in the three study areas (left column) and by LTA in the three global projections (right column). Note the different scales. The error bars indicate the 25th/75th percentiles of 500 simulations for the mussel model and of the range of field harvest within the salinity range for the sugar kelp model (left column).
Nutrient and carbon removal potential in the study area
Nutrient and carbon removal by harvesting of blue mussels and sugar kelp followed the pattern of FW-biomass production (Fig. 4). Carbon removal was assessed here as CO2 incorporated in seaweed biomass through photosynthesis and growth, and in mussel shells by bio-calcification. The CNP removal was highest for mussels in the Baltic Sea due to the high potential biomass yield of small mitigation mussels. The overall potential NPC removal by LTA was highest in the North Sea followed by the Baltic Sea and was lowest in the Danish Straits due to the small allocated OWF area (Table 1). The total NPC removal of LTA farms was 6 Kt-N y−1, 0.4 Kt-P y−1 and 28 Kt-C y−1 (102 Kt-CO2 y1) (Fig. 4).
Global projections of OWF-LTA
In all world regions, current LTA produces mussel species belonging to the family Mytilidae and brown seaweeds and with harvest yields comparable to the species in the study area (Tables 2 and 3). Due to the expected rapid development of OWF and LTA, Northern Europe showed the highest potential for OWF-LTA. Globally, the realistic projection of OWF-LTA by the year 2030 estimated a LTA production of 24,487 Kt-FW y−1, which is equivalent to 132% of the current LTA production worldwide (Table 2). The pessimistic and optimistic projections of OWF-LTA production were 22% and 400% of the current levels, respectively. The realistic global projection predicted a total nutrient and carbon uptake and removal potential at 285 Kt-N y−1, 17 Kt-P y−1 and 1160 Kt-C y−1 (4,226 Kt-CO2 y−1) (Fig. 4).
Environmental variables were extracted from all the projected OWF sites in each region for a comparison with growth conditions in Northen Europe (Fig. 5). Salinity was >16 psu at the OWF sites in all regions except for Northern Europe, due to the influence of the brackish Baltic Sea, and a few sites in Southern Europe and Asia (Fig. 5a). Compared to Northern Europe, the largest deviations of environmental variables were found for Southern America and Africa, showing higher temperatures, lower Chl a concentrations, lower NO3- concentrations, and higher flow velocities (Fig. 5b–e). Flow velocity is important for the nutrient and food supply to the farms to avoid limitation of LTA growth. The critical flow velocity ratios (only mussels) were in most cases above the threshold of 0.5 indicating that food limitation of mussel culture was not a problem (Fig. 5f). Southern Europe showed the lowest critical flow velocity ratio due to a combination of low Chl a concentrations and low flow velocity.
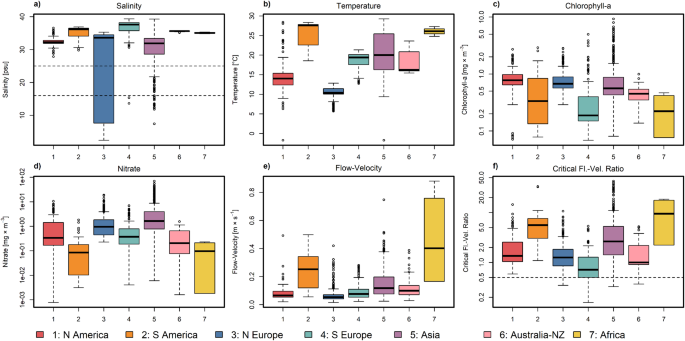
Data was extracted for the future global OWF sites showing (a) salinity, (b) temperature, (c) Chl a concentrations, (d) NO3 concentrations, (e) flow velocity, and (f) critical flow velocity ratio. The box plots show the median, 25th and 75th percentiles (boxes), the full range of (non-outlier) values as whiskers, and outliers as dots. The fill color of each box represents the different regions. Note: log-scale on y-axis for (c) Chl (a, d) NO3 and (f) critical flow velocity ratio.
Discussion
Innovation combined with holistic and integrated approaches results in rewarding opportunities to accelerate sustainable development and restore the health and integrity of our oceans5,16,42,43,44. The present study demonstrated a great potential for such an innovation; multi-use of OWF-LTA in the North Sea - Baltic Sea transition zone to promote optimal and more sustainable use of marine space (Table 1, Fig. 2). The experiences from this region were used to make regional and global projections of OWF-LTA to highlight the global potential and advantages of multi-use of ocean space and discuss the needed developments for future offshore multi-use to deliver ESS. The three categories of ESS are provisioning, regulating and maintenance, and cultural ESS12.
In the brackish parts of the study area, reduced salinity did not allow the full production potential of commercial mussel culture or sugar kelp to be realized (Fig. 2). However, LTA can be used as a tool to remove nutrients (mitigation culture) at low salinities in eutrophic waters instead of production for consumption23,34,35, when excess nutrients of human origin negatively affect the ecosystem. The harvested mitigation mussels can be used as feed ingredients for fish, pigs, or poultry substituting fish meal or providing spat for mussel culture elsewhere23,45,46. If the mussels are commercially produced for human consumption (salinity >16 psu), the areal efficiency (16–18 t-FW ha−1 y−1) was similar to that from the OWF in the Exclusive Economic Zones (EEZ) of the German Bight (Northern Europe) with yields of 24 t-FW ha−1 y−1 47,48,49. Reported mussel harvest yields from other regions of the world varied from 5 t-FW ha−1 y−1 in Canada to 494 t-FW ha−1 y−1 in Chile (Table 3). The lowest value from Canada is due to low water depth and extra-long distance between the mussel long-lines compared to the other sites, due to operational reasons. Hence, our estimate is conservative when used for the global upscaling.
The production potential of sugar kelp used in the current study (16–18 t-FW ha−1 y−1 in saline areas) was lower than reported for the nearby North Sea EEZ of the German Bight due to lower growth rates and less efficient production methods in our pilot4. In the German Bight, the estimated harvest value was around 30 t-FW ha−1 y−1 31,47,48. For other regions, sugar kelp and similar brown seaweed species are cultured (Table 2) and reported yields varied from 16 t-FW ha−1 y−1 in the U.S. to 124 t-FW ha−1 y−1 in Chile (Table 3). Hence, the modeled value of sugar kelp production should be considered as conservative in the global upscaling4. Harvested sugar kelp can be used directly for human consumption, for probiotic animal feed, or for hydrocolloids used in food and pharmaceutical products18. Expanding the market for human consumption would require a major shift in people's diet towards increased seafood consumption. This transition could be facilitated by the younger generations and may provide a sustainable food production option to a growing world population9,11.
In the North Sea-Baltic Sea transition zone, the reported LTA harvest is currently around 22 Kt-FW of blue mussels (Denmark, Germany, and Sweden)50 and 16 t-FW of sugar kelp in the Danish coastal areas (Danish Fisheries Agency database). Hence, the co-location with OWF constitutes a massive 26-fold expansion potential for LTA in the study area. The potential for multi-use may be even higher if we assume that a larger area (>10%) of the OWF is allocated to LTA5. However, this requires integrated multi-use management to assure that all operations of the OWF-LTA are safe.
The ambitious OWF development in the study area combined with LTA multi-use is a pioneering approach that can be transferred to other regions worldwide with existing or projected OWF, selecting the most suitable sites and local species for cultivation (Table 2). Global projections for seven regions showed that current marine LTA production could be increased by 132% in the realistic projection and up to 400% in the optimistic projection (Table 2). Northern Europe showed the highest OWF-LTA development potential among the regions. The uncertainty of the estimates is lowest in this region due to the overlap with the study area, whereas there may be local environmental and species-specific conditions not considered in the other regions. The higher temperatures in other regions compared to Northern Europe is not considered to be a major problem (Fig. 5b), since seaweed and mussel cultivation is already taking place with locally adapted species (Tables 2 and 3). In the context of global warming, offshore areas may be cooler than shallow coastal waters and farm units can be lowered to deeper and colder waters offshore. Food limitation for mussel farming seemed not to be a problem in the majority of OWF areas according to the critical flow velocity ratio (Fig. 5f). The highest uncertainty for OWF-LTA is in Africa due to few planned OWF sites, limited experience with LTA, and high variability in environmental data (Fig. 5). In Australia-NZ, there is currently no cultivation of brown seaweed (e.g., Undaria pinnatifida, Macrocystis pyrifera, Ecklonia radiata) although it should be possible according to the environmental conditions. Overall, the OWF-LTA approach could contribute to solving the food security issues (SDG 2) and other provisioning services in many regions globally while safeguarding sensitive coastal ecosystems and optimizing the use of marine space in confined offshore blue economy areas (SDGs 7, 12, 13, 14, 17).
The OWF-LTA potentially contributes to regulating the marine water quality by taking up considerable amounts of NPC from the marine environment. During growth, blue mussels incorporate nutrients and C into their biomass by ingestion of phytoplankton and detritus as well as uptake/release of inorganic carbon during bio-calcification of the shell15. Where the ability of mussels to remove nutrients from marine ecosystems is well documented51, the role of mussels in carbon sequestration (defined as the secure storage of carbon-containing molecules for >100 y52) is still uncertain. The potential sequestration depends on a delicate balance between shell formation of calcium carbonate, CO2 release during bio-calcification, and respiration53,54. In this study, we included carbon sequestering in mussel shells assuming that the amount of entrapped CO2 is effectively and permanently removed from the atmosphere and the sea by harvesting and subsequent utilization as building material or deposition as e.g., landfills, representing long-term carbon sinks15,53,55. Sugar kelp assimilates N, P, and CO2 from the water for photosynthesis and growth. It is estimated that approximately 11% of the C fixed during primary production is lost as dissolved or particulate C and exported to deeper waters, where it can be considered sequestered56. In our budgets, however, we did not consider the potential of carbon sequestration by seaweeds, but only accounted for the actual C stored in and removed with the harvested seaweed. Hence, upon the harvesting of mussels and sugar kelp, the nutrients and carbon are removed from the marine system and made available to the bioeconomic system on land, in this way constituting an emission capture and utilization technology, rather than an emission capture and storage technology13. The estimated C capture and removal potential in our OWF-LTA scenario amount to 102 Kt-CO2 y−1 in the study area, corresponding to 40% of the CO2 emissions from the Danish agricultural sector in 2020 (254 Kt-CO2)57. The potential mitigation of climate change through greenhouse gas reductions from this C removal, is only realized in cases where (i) the downstream LTA value chain emissions are lower than the net negative emission at the time of harvest4, (ii) the LTA substitutes an ingredient or product with a higher carbon footprint9, or (iii) the seaweed contains bioactive components which reduce ruminant enteric fermentation, and hence methane emission from cattle production58,59. Globally, estimated C capture and removal was up to 4226 Kt-CO2 by OWF-LTA in the realistic scenario (SDG 13).
Locally, LTA acts as an ecological engineering tool for mitigating eutrophication effects43 and could remove a total of 6 Kt-N y−1 and 0.4 Kt-P y−1 in the study area (Fig. 4). When considering the Danish Straits and the western Baltic Sea, this corresponds to 3% and 2% of the regional reduction of N and P, respectively, needed for fulfilling national obligations concerning the EU Marine Strategy Framework Directive as specified in the Baltic Sea Action Plan60. Hence, LTA could support land-based eutrophication mitigation measures and accelerate the required nutrient reductions (SDG 14)35. Co-producing mussels and sugar kelp in an integrated multi-trophic aquaculture solution allows for more stable ESS provision over different seasons as mussels take up more nutrients from June to November61 and sugar kelp from October to May4,61. When overlapping in space and time, inorganic nutrients excreted by mussels can be utilized by the sugar kelp62.
The regulating ESS of LTA also manifests their positive effect on marine water quality as increased water clarity, lower Chl a concentration, lower sedimentation, and increased oxygen concentration on basin-scale18,35,63,64. In addition, seaweed culture produce oxygen and increase pH locally18. When increasing the extent and density of LTA, the ecological carrying capacity issues should also be considered to avoid unacceptable changes to the ecosystems65,66,67,68. Extensive mussel farms can potentially make plankton food unavailable to other nature values with potentially negative consequences for both ecosystems and fishery69,70. Further, sedimentation of mussel bio-deposits can cause accumulation of organic matter underneath the farms leading to local hypoxia, inhibition of denitrification, and increased nutrient fluxes71,72,73. Large-scale seaweed production has been suggested to compete with phytoplankton for nutrients, and thus potentially limit pelagic primary production17,74. However, these negative scenarios are very unlikely to happen in the coming decades and centuries as nutrient loads are still excessive from terrestrial ecosystems and the quantity of legacy nutrients that are stored in the marine ecosystems represents an almost unlimited supply17. Local hypoxia under mussel farms will only manifest under very sheltered conditions but these conditions also represent poor mussel growth areas75. Moreover, offshore areas have strong currents diluting the negative effects of sedimentation from LTA76. The carrying capacity of any LTA solutions can be estimated by 3D modeling and must be site-specific due to the interactions with local environmental conditions68,70. However, in other offshore areas, nutrient concentrations can become relatively low, and the advantage of OWF-LTA may be less obvious considering the negative effects (e.g., fuel) from longer transportation time.
Technology and regulatory development is needed for adapting LTA infrastructure to the more exposed sites and avoiding physical damage to the infrastructure, loss of cultured biomass and at the same time ensuring full safety of operation in OWF5, to name a few. Predation on mussels by eiders can also cause substantial loss of biomass and respective protection measures should be further developed and tested77. Offshore, mussel spat transfer from coastal farms to the OWF may be needed due to limited natural settling34. However, this in turn poses the problem of facilitating the transfer of epiphytic species, parasites, and diseases from nearshore to far-shore water bodies, which in turn may enable a negative impact on the ecosystem in the OWF78,79. The introduction of foundations and farm structures as artificial hard substrates may facilitate the spreading of non-indigenous species, some potentially invasive, in a stepping-stone effect80. Further, optimization of infrastructure design for seaweed cultivation focused on offshore cultivation, selective breeding of sugar kelp towards cultivars with optimized biomass yield at lower salinity, and exploration of other seaweed species such as Ulva could increase the seaweed harvest potential in the Baltic Sea33,81.
The social carrying capacity is the amount of aquaculture activity that can take place in one specific area without adverse social impacts67. Hence, detailed spatial planning of multi-use can initiate discussions with stakeholders, including the OWF operators, and should be an important part of ecosystem-based management24,65,82. It is anticipated, however, that the level of conflicts will be lower offshore due to fewer users, a longer distance to land, and a higher ecological carrying capacity. However, fish trawling will probably be banned in the OWF areas and potentially substituted by angling and passive fishing.
The economic carrying capacity of expanding the production and market of LTA products must also be considered, as increasing the landings will likely negatively influence the price of blue mussels and sugar kelp on the global market. Further, the individual business plans of LTA in the OWF must be viable considering the market value of the end products, the longer transports from land increasing fuel, and potential sharing of operational costs (SDG 17)11. Generally, the regenerative nature of LTA enables the simultaneous delivery of ESS of both a provisioning and a regulating/maintenance nature and has a considerably lower carbon footprint than terrestrial bio-based products9,14. The extent to which the climate/environmental footprint of the LTA will increase due to longer transportation to offshore locations, or sharing of logistics and infrastructure (e.g., sharing of boats and personnel, charging of electric boats, monitoring by robotics) between multiple users will lead to an overall footprint reduction, remains to be tested7,11,17.
Overall, a large potential exists for implementing multi-use of ocean space such as OWF-LTA worldwide supporting the United Nations SDGs, in particular SDG2 (zero hunger), SDG 12 (responsible production and consumption), SDG 13 (Climate action), SGD 14 (Life under water) and SDG 17 (partnership for the goals), framed as "holistic and integrated approaches to sustainable development that will guide humanity to live in harmony with nature and lead to efforts to restore the health and integrity of the Earth's ecosystem"16,83,84.
Methods
Scenario of OWF-LTA multi-use in the study area
The realistic potential of OWF-LTA was estimated in the study area of the North Sea-Baltic Sea transition zone (Fig. 2). Data on OWF locations, names, and areas in the study were mainly obtained from the 4C global offshore database version of January 2023 (accessible via https://map.4coffshore.com/offshorewind/). The two OWFs Vesterhav Nord & Syd (sites 4 and 6) (Fig. 2, Supplementary Table 1) are combined in the 4C global offshore database. However, we used area and location data from EMODnet76, where both are listed individually. This was to consider the large distance and different LTA production characteristics between these two OWF sites. In the 4C global offshore database, there are two OWFs named Kriegers Flak. Therefore, we added corresponding country codes for Denmark (DK) and Sweden (SE) to the names (sites 12 and 25) (Fig. 2, Supplementary Table 1). The OWF we called Nordsøen (3 GW) (site 1) (Fig. 2, Supplementary Table 1), refers to a 3 GW scenario taking into account the areas Nordsøen Tender 1–3 from the 4 C global offshore database, which roughly corresponds to scenario 1a outlined in85. Only wind farms in open marine waters were considered.
In the study area, 31 potential OWF sites could serve for multi-use with LTA in the future: 16 OWF are in operation, one is under construction, eight are approved and six are planned in the three countries Denmark, Sweden, and Germany (Fig. 2, Supplementary Table 1). The newly planned OWF projects by the Danish National Authorities to be realized before 2030 are in the North Sea (sites 1 and 2), the Danish Straits (site 9), and the Baltic Sea (sites 26, 30, and 31) (Fig. 2, Supplementary Table 1). The OWFs are located across a salinity gradient from 34.5 psu in the North Sea to 7.8 psu in the Baltic Sea (Supplementary Table 1). We divided the study area into three sub-areas according to salinity; the North Sea with salinities above 25 psu (sites 1–7), the Danish Straits with salinities from 16 to 25 psu (sites 8–14), and the Baltic Sea with salinities below 16 psu (sites 15 to 31) indicating the threshold for reduced growth of blue mussels and sugar kelp86,87. The multi-use scenario applied in this study was defined as a total of 10% of the OWF area dedicated to LTA. This is a conservative estimate and corresponds to scenarios applied in the German and Dutch parts of the North Sea88,89. The main criteria for the 10% was the available space within the wind park, which is limited by wind turbine foundations, scour protections, underwater cables, service routes, and associated safety zones41. Within the 10% of each OWF area dedicated to LTA, a 50/50 areal distribution between the production of mussels and seaweeds was assumed. The aquaculture period was assumed to be from June to November for mussels which are optimal for mitigation culture61 and from October to May for seaweeds4 in northern Europe.
Background data for LTA production models
We used publicly available Copernicus data products based on numerical models and satellite observations to derive spatial models of monthly aggregated salinity, temperature and horizontal flow velocity90,91 in the upper 10 m water column, and surface Chl a concentrations92,93. These products have different spatial focus areas addressing global scale80, the Atlantic Ocean92, or the Baltic Sea91,93. For each product, we first calculated means and standard deviations for each month of the year and the time range from 2007 to 2017, while Chl a concentrations were log-transformed beforehand. The years were selected to obtain comparability with a former spatial modeling study on blue mussel mitigation farms94. The resulting layers were then projected onto a 500 × 500 m grid using bilinear interpolation. To account for the spatial focus area of each dataset, we used weighted averaging across the overlap region in the western Baltic Sea using the overall average salinity, S, for each pixel to determine the weights:
Here, wAO is the weight attributed to the datasets with either global90 or Atlantic Ocean92 focus area, while wBS is the weight attributed to the datasets with Baltic Sea91,93 focus area. The weight functions describe sigmoid curves between boundary salinities of 10 psu, respectively 25 psu always summing up to 1. All described calculations were performed in 'R' (v. R-4.1.2) using the Terra library (v. 1.4-22).
Mussel harvest and nutrient removal model
In a previous study94, a modular spatial farm scale model was developed and used to estimate explicit growth potentials and nutrient removal rates of mitigation culture of blue mussels (Mytilus spp.) across the western Baltic Sea. In this model, the harvest of mussels is a function of monthly salinity, temperature, Chl a
Comments
Post a Comment