Water warming increases aggression in a tropical fish | Scientific Reports - Nature.com
Abstract
Our understanding of how projected climatic warming will influence the world's biota remains largely speculative, owing to the many ways in which it can directly and indirectly affect individual phenotypes. Its impact is expected to be especially severe in the tropics, where organisms have evolved in more physically stable conditions relative to temperate ecosystems. Lake Tanganyika (eastern Africa) is one ecosystem experiencing rapid warming, yet our understanding of how its diverse assemblage of endemic species will respond is incomplete. Herein, we conducted a laboratory experiment to assess how anticipated future warming would affect the mirror-elicited aggressive behaviour of Julidochromis ornatus, a common endemic cichlid in Lake Tanganyika. Given linkages that have been established between temperature and individual behaviour in fish and other animals, we hypothesized that water warming would heighten average individual aggression. Our findings support this hypothesis, suggesting the potential for water warming to mediate behavioural phenotypic expression through negative effects associated with individual health (body condition). We ultimately discuss the implications of our findings for efforts aimed at understanding how continued climate warming will affect the ecology of Lake Tanganyika fishes and other tropical ectotherms.
Similar content being viewed by others
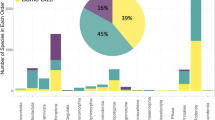
New estimates indicate that males are not larger than females in most mammal species
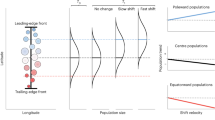
Marine fishes experiencing high-velocity range shifts may not be climate change winners
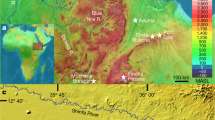
Adaptive foraging behaviours in the Horn of Africa during Toba supereruption
Introduction
Human-induced rapid environmental change (HIREC)1 has altered species interactions and caused species range shifts, leading to population declines in both aquatic and terrestrial ecosystems2,3,4,3.0.CO;2-G (1997)." href="https://www.nature.com/articles/s41598-020-76780-1#ref-CR5" id="ref-link-section-d39241094e609_3">5,6,7. Novel physical conditions caused by HIREC, which exert selective pressures that have not been previously encountered during an organism's evolutionary history, are expected to be especially detrimental to species persistence1,8,9. While the literature is rich with examples of how novel selection pressures caused by HIREC, including habitat destruction10,11, pollution12, over-exploitation13,14, and species invasions15,16 have caused population declines, we are only beginning to fully appreciate the impact of climate-driven changes to the environment.
Our expectation is that the impact of climate change will vary among the world's biomes, with species that have evolved in thermally stable, tropical environments being more vulnerable to climate change than temperate species that have evolved in more thermally variable environments17,18,19. In this way, the conventional wisdom is that even small changes in the climatic regime (e.g., warming) would have large effects on the species that inhabit coral reef, river, and forest ecosystems in the tropics4,9,20,21,22. Furthermore, changes in the thermal environment are expected to affect the demographics of native populations through direct and indirect influences associated with altered physiology (e.g., metabolism)23,24.
For aquatic ectotherms such as fish residing in tropical lakes, warming could be problematic for at least two reasons. First, increased water temperature is expected to raise basal metabolic rates, which might push these organisms past their optimal thermal window18. In turn, their need to acquire more energy to survive, grow, and reproduce would be expected to increase25,26,27. Second, because warming can alter the thermal structure of lakes such that primary production decreases28,29,30, insufficient energy may be available to support the production of prey needed to counterbalance the expected increase in metabolic demand.
While species can and have evolved to cope with environmental changes31,32, rapid warming and other forms of HIREC likely will require alternative means for populations to survive under these kinds of selection pressures. Behavioural change offers a mechanism by which individuals could persist in the face of rapid environmental change. For example, individuals may become bolder and more aggressive towards conspecifics as a means to secure food, territorial, and reproductive resources33,34,3.0.CO;2-E (1992)." href="https://www.nature.com/articles/s41598-020-76780-1#ref-CR35" id="ref-link-section-d39241094e716">35. The effect(s) of HIREC on individual behaviour unfortunately remain(s) less understood than other aspects of an organism's phenotype (e.g., physiological responses)36.
Understanding this linkage is important because changes in an individual's behavioural phenotype hold great potential to alter community (food web) interactions, as well as mediate future fitness. Increased boldness or aggression in fish, for instance, has been shown to increase the risk of being preyed upon by higher consumers37, as well as being harvested by human fishers38,39,40. While previous studies have shown that rapid warming can cause an acute (immediate) increase in aggression in ectotherms such as fish3.0.CO;2-E (1992)." href="https://www.nature.com/articles/s41598-020-76780-1#ref-CR35" id="ref-link-section-d39241094e739">35,41,42,43, whether this effect on behavioural phenotype is chronic (long-lasting) remains unclear, owing to the potential for acclimation.
This knowledge gap could be especially important for understanding the dynamics of organisms that form pair bonds or reside in social groups. Pair bonding and group-living are common in many animals, owing to the benefits that they can confer to activities vital to individual fitness, including territory defence44,45, care of young46,47, and safety from predators48,49. Crucially, the stability and reproductive success of social groups, including mating pairs, can be influenced by the behaviour (e.g., aggression) of individuals, as has been shown for the highly social Lake Tanganyika cichlid Neolamprologus pulcher50. Temperature-induced changes in behaviour, therefore, could result in fitness effects through both the direct consequences of individual behaviour (e.g., risk of injury from increased aggression) and indirect impacts of changes in an individual's social context41.
The need for such knowledge is especially evident in Lake Tanganyika, a Great Lake located in eastern Africa. As with other African Rift Lakes, Lake Tanganyika supports a flock of endemic (cichlid) fishes that is being threatened by HIREC, including climate warming51,52,53,54. In addition to potentially raising the metabolic demand of these ectothermic fish27,55, observed rapid increases in surface (epilimnetic) water temperature during recent decades has strengthened thermal stratification28,29,30,56,57,58. In turn, both the upwelling of nutrient-rich bottom waters and vertical mixing of the water column have decreased, resulting in reduced productivity of the lower food web that resides in the epilimnion56,59. Because thermal stratification is expected to only strengthen and last longer with continued warming of epilimnetic waters30, the need to understand how organisms that reside in epilimnetic waters of this ecosystem will respond to continued warming is paramount58,60.
Herein, we conducted a laboratory experiment with an endemic Lake Tanganyika cichlid, Julidochromis ornatus (golden julie), to better understand how anticipated water warming might affect individual behaviours over the long-term within this species and potentially the many other endemic cichlids like it. We chose J. ornatus as our study species because it has a life-history similar to the many other endemic cichlids in East Africa and it is commonly found in the shallow, nearshore areas of Lake Tanganyika, have been vulnerable to water warming56,58,60. Specifically, we exposed J. ornatus adults to one of two thermal conditions: current-day water temperatures (~25.5 \(^\circ\)C)56,61 and the projected water temperature at the end of the century (~29 \(^\circ\)C)56,58, which has already been observed in surface waters of Lake Tanganyika58,61.
To increase realism in our experiment and to assess the potential for long-lasting behavioural change, we used longer initial laboratory acclimation (10–12 months), thermal transitional (2 weeks), and experimental exposure (8 months) periods than other studies of this kind3.0.CO;2-E (1992)." href="https://www.nature.com/articles/s41598-020-76780-1#ref-CR35" id="ref-link-section-d39241094e917">35,41,42,43. We predicted that individuals in the high-temperature (i.e., future climate) treatment would become more aggressive relative to the low-temperature (i.e., control) treatment, because (1) temperature and metabolic rate have been shown to be positively correlated in ectotherms18,62 and (2) other studies with ectotherms have generally shown individual metabolic rate and aggression to be positively related36,37,55,63.
Results
Body size and condition
Differences in body size and condition became evident during the course of the experiment, which needed to be accounted for in our predictive modelling of aggression. No significant differences in mean wet mass (M), total length (TL), and body condition (cond) existed between the low-temperature and high-temperature treatments at the start of the experiment (all \(\chi ^2_{1,102} < 0.6\), \(p>0.4\)). However, significant size differences existed 17 months later, at the experiment's conclusion (for both M and TL: \(\chi ^2_{1,102}>44\), \(p<0.001\)). Specifically, fish grew from a mean (\(\pm 1\) SD) size of \(2.6\pm 1.1\) g and \(57.4\pm 7.6\) mm at ~ 9 months of age to \(3.4\pm 1.4\) g and \(62.3\pm 8.3\) mm at 26 months of age; we reported pooled means across treatments because neither temperature nor its interaction with time (Pre vs. Post) explained a significant amount of variation in M or TL (both \(\chi ^2_{1,102} < 0.6\), \(p>0.3\)). By contrast, a statistically significant change in cond was not observed during the course of the experiment (\(\chi ^2_{1, 102}=0.58\), \(p>0.4\)).
Given that fish size and health have been shown to affect individual behaviour36, as well as the fact that significant somatic growth occurred during the 8 months of experimentation and more than 2-fold individual variation in M and cond was observed within and among treatments (M range = 0.9–5.7 g; cond range = 1.9–4.2), we retained both M and cond as covariates in our aggression analyses. While TL also varied considerably within and among treatments (TL range = 43.2–75.0 mm), we did not include this factor as a potential covariate in our modelling to reduce multicollinearity, given that TL was highly correlated with M (\(r=0.95, p<0.001, df = 100\)).
Aggression
Aggression scores varied widely during the course of the experiment, with a wide range of aggressive displays, both overt and restrained, being observed (Supplementary Fig. S1). Because overt and restrained aggression were significantly positively correlated (\(r = 0.36, p<0.001, df = 100\)), and total aggression was positively correlated with both overt and restrained aggression scores (both \(r > 0.8, p<0.0001, df = 100\)), we used total aggression as our main response variable in subsequent analyses.
Our model selection procedure identified a single predictive model of total aggression as the most robust (AICc = 1125.8; weight = 0.46; Table 1). This model consisted of only temperature (temp; \(F_{1, 101}=14.84\), \(p<0.001\)) and the temperature\(\times\)time interaction (temp:time; \(F_{1,101}=10.80\), \(p<0.01\)) as predictors. However, body condition (cond; \(F_{1, 101}=9.23\), \(p<0.01\)) and test rank (\(F_{1, 101}=4.36\), \(p<0.05\)) were also included in the final model as important covariates (Table 1).
Increased temperature led to increased aggression in our experiment (Table 2; Fig. 1). While total aggression scores did not significantly differ between treatments before the temperature manipulation, total aggression differed between treatments after the temperature manipulation (Table 2; Fig. 1). Specifically, total aggression scores significantly increased for the individuals held in the manipulated (high-temperature) treatment (ranged: ~194 to ~247 in a 10-min trial), whereas it remained unchanged in the individuals held in the control (low-temperature) treatment (Table 2; Fig. 1).
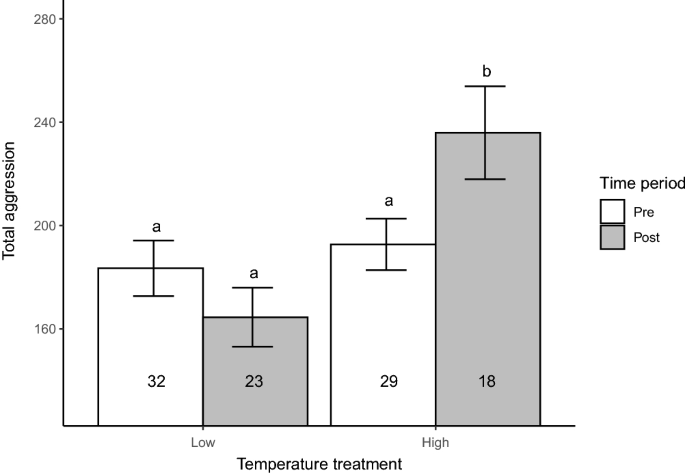
Total aggression scores of Julidochromis ornatus at the start (Pre; measured at 9 months of age) and end (Post; measured at 26 months of age) of our laboratory experiment. While all individuals were subjected to 25.5 \(^\circ\)C (Temperature = Low) prior to the start of the experiment (Time = Pre; 9 to 18 months), temperature was raised to ~29 \(^\circ\)C during 18–26 months of the experiment (Time = Post) in half of the tanks (Temperature = High), whereas it remained unchanged in the other half of the tanks (i.e., Temperature = Low). The mean (bars) and standard errors (error bars) of total aggression in each treatment are presented. Numbers in each bar indicate sample sizes. Treatments sharing a letter do not significantly differ (Tukey-adjusted LSMeans comparisons; \(p < 0.05\)).
While the effect of test rank (order) on aggression was inconsistent in relation to total aggression (Supplementary Fig. S2), the seeming effect of body condition on total aggression was clearer. In general, aggression was inversely related to body condition, as indicated by the negative coefficient (-50.7) associated with cond in our selected predictive model (Table 1). To further illustrate this relationship, we used our most parsimonious model to predict total aggression as a function of body condition in all treatments (Fig. 2). This analysis showed that, even though individuals from the high-temperature treatment had an overall greater mean (\(\pm 1\) SE) condition at the end of the experiment (\(3.0\pm 0.1\)) compared to the individuals in the low-temperature treatment (\(2.7\pm 0.1\)), cond was negatively related to aggression. Thus, individuals in poorer condition were generally more aggressive than those in better condition regardless of treatment (Fig. 2).
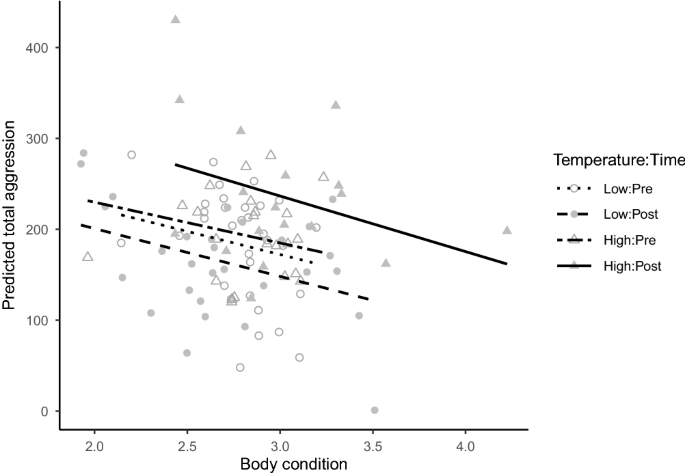
Predicted total aggression scores using the most parsimonious (based on AICc) linear mixed model versus body condition in Julidochromis ornatus individuals used in our laboratory experiment. All individuals were subjected to 25.5 \(^\circ\)C (Temperature = Low) prior to the start of the experiment (Time = Pre; 9 to 18 months). By contrast, temperature was raised to 29 \(^\circ\)C (Temperature = High) during 18–26 months of the experiment (Time = Post) in half of the tanks (Temperature = High), whereas it remained unchanged in the other half of the tanks (i.e., Temperature = Low). The regression line for each treatment is shown.
Discussion
The main objective of our study was to shed insight into how climate warming might affect the behaviour of tropical freshwater ectotherms such as the endemic Lamprologini cichlid assemblage of Lake Tanganyika. Three major findings emerged from our experiment. First, we found that the frequency of aggressive behaviour in the Tanganyikan cichlid Julidochromis ornatus increased after individuals were exposed to the future projected temperature of Lake Tanganyika surface waters at the end of the 21st century (i.e., ~29 \(^\circ\)C)56, which also happens to be the current-day extreme high temperature56,61. The finding is important because the observed increase in aggression with increased water temperature indicates that this behaviour is not canalized with respect to temperature for this species, which may help this (and perhaps other tropical) species persist in the face of continued human-induced rapid environmental change (HIREC). Second, our experiment showed that environmental change such as water warming can modify the behaviour of ectotherms like fish, not only in the short-term as other studies have previously documented3.0.CO;2-E (1992)." href="https://www.nature.com/articles/s41598-020-76780-1#ref-CR35" id="ref-link-section-d39241094e3231">35 but also over the long-term; increased aggression persisted for the duration of our 8-month experiment, which we would expect to have major consequences to individual energy budget, growth, and fitness, as well as community and social interactions. Finally, we unexpectedly found that individual aggression in J. ornatus adults increased as individual body condition (our proxy for health36) declined. Thus, individuals in the poorest condition were more likely to expend additional energy exhibiting aggressive behaviours than those in the best condition. Such behaviour could be viewed as highly risky, with potentially negative consequences for future growth performance and fitness, in the absence of sufficient prey resources to sustain this energetically expensive behaviour55,64,65. Below, we discuss these findings in more detail, as well as the implication of this behavioural shift in ecosystems such as Lake Tanganyika, where climate warming has also been causing reduced production at the base of the food web in shallow surface waters where cichlids such as J. ornatus reside28,29,30,60.
Our experiment clearly demonstrated the ability of environmental warming to increase aggression, a finding that has been documented in other ectotherms, including other fish34, amphibians66, and arachnids67. Currently, however, the proximal mechanism driving this linkage still remains uncertain. For example, we do not know whether this relationship is related to altered physiology (e.g., increased metabolism), behavioural "stress" associated with lost reproductive output, or perhaps just a spurious response associated with another behavioural and (or) physiological change not measured in our experiment.
At the outset of this experiment, we expected aggressive behaviour to increase in our high-temperature treatment, given that temperature and metabolic rate have been shown to be positively correlated in ectotherms18,62 and other studies with ectotherms have demonstrated positive relationships between individual metabolism and aggression36,37,55,63. However, whether environmentally-driven metabolic change can truly drive behavioural change still remains largely theoretical68, which is partly due to the complexity of processes that govern behavioural changes36 and partly due to ambivalent support from the literature. For example, individuals with a fast standard metabolic rate have been shown to be inherently more aggressive and bolder (i.e., have a fast pace-of-life) than conspecifics with a slow standard metabolic rate69. Likewise, contrary results have been found, wherein bolder individuals had a lower standard metabolic rate than less bold ones70.
Although we cannot be certain, we suspect that the increase in aggression in the individuals exposed to the higher temperature was driven by changing metabolic demands. Similar to other fish taxa71,72,73,74, as well as other ectotherms75,76,77,78, metabolic rate has been shown to increase with increasing temperature in J. ornatus27 and other cichlids55. For example, using a similar approach to assess aggression as our own, overt aggressive behaviours associated with locomotion (e.g., biting, tail-slapping) in Oreochromis mossambicus, another African cichlid, were found to be positively correlated with metabolic rate55. Furthermore, in a study conducted with a small subset of adult males from our study population (n = 8 and n = 10 in the low- and high-temperature treatments, respectively), those individuals exposed to the elevated temperature (29 \(^\circ\)C) appeared incapable of physiologically acclimating, as their routine metabolic rate significantly increased relative to the pre-manipulation period and also was significantly higher than those individuals in the control treatment (25 \(^\circ\)C), after 6 months of exposure to the temperature manipulation27. Additionally, the adult males in the high-temperature treatment did not gain (or lose) body mass, whereas individuals in the low-temperature treatment gained mass, with breeding pairs associated with males in the high-temperature treatment experiencing a significantly greater reduction in their mean reproductive rate (e.g., average number of broods produced per pair per day)27.
While we do know that mass-specific metabolic rates did not differ among our study individuals across our four recirculating systems at the outset of experiment (i.e., pre-temperature manipulation; R.M.B. and S.A.L., unpublished data), insufficient metabolic data existed at the end of the experiment to allow us to determine the role of metabolic change in driving aggression. Thus, we do not know if these results are generalizable to our (broader) study population. Given this uncertainty, more work is needed to help us better understand whether, for example, increased metabolic rate itself, hunger associated with increased metabolic needs, some other physiological change such as altered stress hormone levels, lost reproductive fitness, or their combination underlies the long-lasting effect that increased temperature had on aggression. In addition to having the metabolic data, knowing the time-course of aggression (i.e., when it first emerged) and stress levels of fish (e.g., corticosteroid levels)79 would have been helpful in delineating the proximal mechanism(s).
Even though aggression in African cichlids like Julidochromis ornatus is common in nature80,81, our observed long-lasting increase in aggression suggests that this behaviour is not canalized. This finding is important as it indicates the potential for this behavioural adjustment to help buffer this species, and perhaps others like it, against environmental change such as water warming. The ability to become more aggressive might allow individuals to better protect and obtain resources82,83,84, which may become limiting in Lake Tanganyika, if climate change continues to reduce primary production in shallow surface waters28,29,30. Heightened aggression could allow organisms to take over and control better territories, which could provide enhanced access to resources85,86,87 that can benefit both growth88 and survival89. We would also expect more aggressive individuals to secure food more readily than their less-aggressive counterparts, perhaps allowing the aggressors to outgrow and outcompete other individuals90,91,92,93. If true, innately aggressive individuals, as well as those with an ability to adjust their behaviour to become more aggressive, might be expected to have a fitness advantage over non-aggressive individuals and those that lack behavioural plasticity in tropical ecosystems like Lake Tanganyika, which are experiencing climate-driven water warming and reduced production at the base of the food web28,29,58.
In reality, however, such potential behavioural benefits are more nuanced. Heightened aggression, for example, could expose individuals to a higher risk of mortality from predators83,94. Likewise, while we would expect the more dominant and aggressive individuals to control better breeding shelters in nature, the corresponding rewards of territory control are likely to depend on the territory's function in conjunction with individual's general foraging habits. Aggressive individuals in some species (e.g., J. ornatus) may still struggle to find sufficient food resources to sustain their heightened metabolism and activity level, if their breeding territory does not also help them secure food resources. In this way, the heightened, energetically expensive55,64,65 aggressive behaviours displayed by individuals in poorer condition (health) could exacerbate the negative impacts of temperature increase. Because our study design did not allow us to explore intraspecific interactions, we see value in continued laboratory and field-based research endeavors aimed at determining whether aggression serves as a net benefit or cost to individuals under increased thermal regimes. Equally as interesting would be learning how size, sex, and body condition interact to affect the acquisition of resources such as breeding territories and food under different thermal regimes. The need to understand the costs and benefits of temperature-driven increases in aggression is especially important for Lamprologini cichlids, given that climate change has been interacting with other forms of HIREC in Lake Tanganyika to reducing production at the base of the lake's food web28,29,30.
Although exceptions exist95, exposure to food shortages have been shown to generally increase aggression in other species38,82,86,96,97,98, including ectotherms93,99. Thus, our finding that surviving pairs in our high-temperature treatment were generally more aggressive than those in our low-temperature treatment is supported by the literature and hints at behavioural adjustment or plasticity as a means to cope with stress (e.g., potential food shortages) caused by water warming or other forms of HIREC. While our experiment showed that exposure to a constant long-term temperature increase can lead to long-term behavioural change, what remains unclear is how the timing, duration, and magnitude of warming and its discontents influence the onset and persistence of aggressive behaviour. Similarly, we cannot be certain as to how daily fluctuations in temperature, which are typical of all ecosystems, including tropical ones such as Lake Tanganyika28,56, would affect the persistence and intensity of aggression in ectotherms such as J. ornatus. We did, however, find that unintended short-term temperature fluctuations (increases) in our control treatment towards the end of the experiment (Supplemental Fig. S3) did not cause corresponding increases in aggressive behaviour in our study individuals. This finding supports our hypothesis that aggressive behaviour is driven more by long-term thermal fluctuations (months) than short-term ones (days to weeks). In our experiment, while the low temperature treatment group experienced an unintended temperature fluctuation briefly close to the end of the experiment, we did not observe a corresponding increase in aggressive behaviour in these fish. Clearly, answers to these questions will be necessary to better understand the response of wild populations of this and other cichlid species.
While our experiment explicitly tested individual fish behavioural responses to an artificial image of itself (similar size and reactions), which some have argued does not fully represent fish aggression perfectly100,101,102, aggressive intra-pair interactions do occur, with the larger individual typically being more dominant (Authors, personal observations). Because Julidochromis ornatus do not exhibit obvious sexual dimorphism (e.g., colour differences, mating tubercles), body size is an important determinant of parental behaviour. These observations correspond with natural observations of aggressive behaviours in J. ornatus where aggression is often targeted at conspecifics with the larger individuals generally being the aggressor103. When in breeding pairs, larger individuals tend to dominate and coerce their smaller partner into providing more parental care60,80. Hence, we would expect heightened aggression directed at a mate to lead to reduced fitness via mate mortality and reduced reproductive output. This notion raises the interesting question of whether the reduced reproductive output observed in a small subset of our study individuals exposed to a high temperature27 was a direct response of food limitation (i.e., a metabolic response), heightened aggression and competition for food (i.e., behavioural response), or both. Clearly, more work is needed to delineate the relative importance of these potential mechanisms.
In larger breeding groups with polygamy and cooperative breeding, the effect of temperature on behaviour would require further study, owing to the inherent complexities of sociality. For example, a similar study investigating temperature effects on fish behaviour, but in a social context, also observed a similar temperature-aggression relationship to our own41. Interestingly, the authors also found that subordinate individuals had lowered growth rates under higher temperature relative to the dominant individuals, which were unaffected41. Findings such as these highlight the need to explore how temperature-driven increases in aggression might affect social interactions, group structure, and reproductive output in J. ornatus and other cooperatively breeding species.
In conclusion, we cannot pretend that our simple, artificial experiment can allow us to predict with any certainty how continued warming and all of its discontents (e.g., reduced primary production)28,29,30 will drive behavioural change and fitness in tropical ectotherms such as J. ornatus in the wild. Even so, we do feel that our experiment offers important, novel insights that provide an excellent basis for future laboratory- and field-based research. Specifically, our findings indicate the potential for some tropical species to cope with warming and perhaps other forms of HIREC28,29,30 by adjusting their behaviour. To this end, we showed that individual aggressiveness in J. ornatus, a common cichlid endemic to Lake Tanganyika, increased when exposed to the warmer water temperature projected for Lake Tanganyika at the end of the 21st century, with this behavioural adjustment being long-lasting (i.e., for the entirety of our 8-month experiment). Furthermore, our experiment offered a clue into the proximal mechanism underlying this behavioural shift as the most pronounced increases in aggression occurred in individuals in the poorest body condition (health). Knowing that long-lasting, climate-driven behavioural adjustments might be possible offers some optimism, given that tropical species are viewed as being especially vulnerable to climatic warming4,9,20,21,22. For example, the ability to become more aggressive could allow this species, as well as other endemic African cichlids with similar life-histories, to cope with the immediate effects of warming by improving access to critical resources such as breeding shelters and food85,86,87,88,89. Importantly, this temperature-induced increase in aggression may also come with costs, including increased predation risk or increased mate aggression that could potentially undermine reproductive success.
Given these tradeoffs, we are excited by the potential of future research endeavors aimed at determining whether heightened aggression serves as a net benefit or cost to individuals under increased thermal regimes. This question seems especially interesting in ecosystems such as Lake Tanganyika, which are also experiencing reduced energy production at the base of the food web due to climate warming28,29,30. Learning how water warming interacts with reduced food availability to drive individual behaviour, and in turn social and food web interactions, is an area in need of research, which could greatly benefit efforts to conserve the many unique species found in tropical ecosystems like the African Rift Lakes. Likewise, insight into whether environmentally-driven behavioural adjustments (e.g., increased aggression in response to warming) are truly adaptive and can be passed onto new generations through phenotypic selection and (or) temperature-induced epigenetic effects18,19, which has been implied in other non-behavioural studies104,105, seems especially important for understanding the ability of tropical organisms to cope with novel environmental change. Finally, owing to the many forms of HIREC that are simultaneously affecting the world's aquatic and terrestrial ecosystems1,3,7,106, we encourage research that identifies linkages among environmental conditions, individual physiology, morphology, and behaviour36. Such knowledge could provide a much-needed platform to help us predict the response of broader population and social dynamics to independent and interacting forms of human-driven environmental change.
Methods
Study system and species
Lake Tanganyika is one of the oldest and largest freshwater lakes in the world, supporting approximately 10 million people from Burundi, the Democratic Republic of Congo (DRC), Tanzania, and Zambia59. During the past 1,500 years, Lake Tanganyika has had a stable climate with average temperatures remaining relatively constant until the early 1900s56. Since this time, temperature variation has increased (ranging 23.3 and 28.8 \(^\circ\)C)61, with the average temperature in both shallow and deeper regions of the lake also increasing28,30,56. While water temperatures in deep (> 100 m depth contour), relatively unproductive regions of the lake only increased by 0.2–0.3 \(^\circ\)C during the past century28,30, the rate of increase in shallower, historically productive waters has been about 1 \(^\circ\)C per 25 years since the mid-1960s, which approximates observed increases in air temperature56. Associated with these changes has been reduced primary production at the base of the food web, which is expected to limit food availability to secondary and tertiary consumers such as fish28,29,58.
Owing to its large size, great depth, old age, and historically stable tropical climate, Lake Tanganyika supports hundreds of endemic species, including fishes in the family Cichlidae28,56,59,107,108. Many species of these cichlids and the larger predator populations that they support are vital to the livelihoods of people in this region of the world as both a source of protein and a source of income59,109,110.
Our study species, Julidochromis ornatus, is a substrate-brooding cichlid endemic to Lake Tanganyika. J. ornatus individuals generally inhabit rocky shorelines in both the northern and southern sections of the lake60,80. J. ornatus exhibits substantial variation in its breeding (i.e., mating and social) system, which includes monogamy, polygamy, and cooperative breeding60,80,111. Even so, the formation of monogamous breeding pairs (i.e., pair-bonds) is the most common, occurring in the majority of shallow-water breeding groups and being twice as frequent as the next most observed breeding system60. Given that J. ornatus is commonly found in shallow areas of Lake Tanganyika, where water warming has been occurring rapidly since the mid-1960s56, and it is ecologically similar to many other small substrate-brooding cichlid species throughout the tropics60, we view it as a good indicator species for the many other cichlids inhabiting the lake.
Experimental setup
The individuals used in our laboratory experiment were three generations removed from Lake Tanganyika. We received 200 small juveniles (about 3 months of age) from Reserve Stock Cichlids (East Chatham, NY, USA). During the setup phase of the experiment, the fish were housed at higher densities in 57-L glass aquaria, each containing multiple breeding shelters made of two 7 cm \(\times\) 7 cm slate tiles. As part of a larger experiment investigating fish responses, such as metabolic rate, reproductive life-history to climate warming, we allowed these individuals to form pair-bonds (\(n=72\) male-female pairs) on their own, which happened between 6–8 months of age. Once a pair-bond was formed, we randomly assigned that pair to a 57-L aquarium that had crushed coral substrate (CaribSea Florida Crushed Coral, Fort Pierce, FL, USA) and a single sla...
Comments
Post a Comment