NPF activates a specific NPF receptor and regulates food intake in Pacific abalone Haliotis discus hannai | Scientific Reports - Nature.com
Abstract
Neuropeptides function through G protein-coupled receptors (GPCRs) with high specificity, implying a significant degree of neuropeptide-GPCR coevolution. However, potential neuropeptide signaling systems in non-chordates are relatively elusive. We determined the specificity of the neuropeptide F (Hdh-NPF) signaling system with a cognate receptor (Hdh-NPFR) in the Pacific abalone, Haliotis discus hannai. Phylogenetic and exon–intron arrangement analyses of bilaterian NPF and the chordate ortholog NPY with their receptor sequences revealed a likely common ancestor, and Hdh-NPFR was similar to the NPYR2 subtype among the NPYR1, NPYR2, and NPYR5 subtypes. Among four Hdh-NPFR-related receptors, Hdh-NPFR specifically responded to Hdh-NPF peptide, supported by the dose–response luciferase reporter curve, intracellular Ca2+ mobilization, and phosphorylation of ERK1/2 and its inhibition with a protein kinase C inhibitor. Peptide fragmentations and shuffling of Hdh-NPF with human NPY could not activate the cellular response of Hdh-NPFR. Three-dimensional in silico modeling suggested that interaction of Hdh-NPF C-terminal amino acids with the extracellular loops of Hdh-NPFR is critical for Hdh-NPFR activation. In vivo injection of Hdh-NPF peptide increased food consumption, and knockdown of Hdh-NPF expression decreased food consumption in Pacific abalone. These findings provide evidence for co-evolution of the NPF/Y ligand-receptor system, enabling further research on mollusk orexigenic neuropeptides.
Introduction
Neuropeptides (NPs) synthesized and secreted by neurons are essential regulators in diverse animal phyla. NPs exert their effects locally as hormones, neurotransmitters, and neuromodulators for various physiological functions, including growth, metabolism, and reproduction1,2,3. The evolutionary origins of NPs have been traced to the common ancestor of protostomes and deuterostomes, in which two rounds of genome duplication in the vertebrate lineage gave rise to an expanded repertoire of NP signaling systems with cognate receptors4. For example, identification of the [LV]Wamide ([LeuVal]Trp-NH2) cluster in the eumetazoan species has shed light on the complex mechanism of duplication and gene loss for the origin of these NP families, such as the bilaterian adipokinetic hormone (AKH) and gonadotropin-releasing hormone (GnRH)/corazonin (CRZ) families5. Given the conserved role of GnRH in reproductive endocrine systems, lineage-specific loss of CRZ signaling in vertebrates and duplication of the GnRH signaling system with AKH in invertebrates may account for the global phylogenetic distribution of AKH/CRZ/GnRH-type receptor pathways6.
Neuropeptide Y (NPY), a 36-amino acid (aa) peptide originally isolated from the porcine brain7, belongs to the NPY family of biologically active peptides, together with two other members: peptide YY (PYY) and pancreatic polypeptide (PP)8. In vertebrates, NPY plays an essential role in diverse physiological functions, including food intake, energy homeostasis, anxiety, and stress responses, through interaction with NPY receptors (NPYRs)9,10,11, which belong to class A G-protein coupled receptors (GPCRs), activated by the closely related peptides NPY, PYY, and PP12. Activation of vertebrate NPYRs by NPY primarily results in decreased cyclic adenosine monophosphate (cAMP) accumulation through Gi protein, leading to the inhibition of adenylyl cyclase in mammalian cells12. Furthermore, human NPY-activated NPYRs induced increases in intracellular Ca2+ levels possibly through Gq protein13,14. Recognition experiments of mammalian NPY by its receptors using chemically modified NPYs, receptor mutagenesis, and receptor chimeras demonstrated that NPYRs require both ends of NPY for binding, and intact Tyr-32, Arg-35, and Tyr-36-NH2 residues are critical for the fully active form15,16. Small-molecule compounds that regulate NPY signaling systems have pharmaco-therapeutic implications, with potential efficacy in the treatment of a wide range of metabolic and psychiatric disorders17,18.
Invertebrate NPF, an ortholog of human NPY terminating in a Phe-amide, and NPF-related NPs (e.g., sNPF, FLP-34, and PrRP) exert their effects on target cells by binding to and activating specific types of receptors, leading to changes in the activity of downstream effectors19,20,21,22. Since discovery of the first invertebrate NPF in the tapeworm Monieza expansa23, several NPF orthologs that typically display an RPRF-NH2 C-terminal sequence and a length ranging from 36 to 40 aa have been identified from diverse invertebrate phyla20. However, functional studies for NPF signaling systems with NPFRs and their related receptors have mainly focused on model organisms, especially Drosophila and Caenorhabditis19,20,21. Invertebrate NPF systems, including sNPF, seem to play crucial roles in the coordination of feeding, energy metabolism, circadian rhythm, and reproduction20,24,25,26. Recent findings offer new insights into the roles of the Drosophila NPF signaling pathway in male courtship and germline stem cell proliferation27,28.
Mollusca is the second-largest phylum of living animals, with an estimated 85,000 extant species of mollusks comprising approximately 23% of all named marine animals29. Although the first functional invertebrate NPFR was characterized from the brain of the snail Lymnaea stagnalis30, there is limited and controversial information on the in vivo effects of molluscan NPFs. Injection of NPF increased the filtration rates of clams (Ruditapes philippinarum)31, whereas Aplysia californica NPF was found to reduce food intake in a dose-dependent manner32. Further, administration of L. stagnalis NPF to the snail did not have short-term effects on food intake, but reduced growth and reproductive performance33,34. To fully explain these elusive NPF functions, it is essential to investigate the distribution, spatiotemporal expression pattern, and functional characterization of NPF signaling systems in non-model mollusks. Since the L. stagnalis NPFR is the only known functional receptor interacting with molluscan NPF identified to date30, characterization of additional receptors is needed to better understand the possible roles of NPF in mollusks.
Abalone (Mollusca: Gastropoda: Haliotidae) farms provide high-quality seafood for humans. Recently, farm production of this species has increased from negligible quantities, with the vast majority being produced in China and South Korea at 87% and 10% respectively, according to worldwide production records35. However, environmental and farm stress imposes changes to abalone physiology and metabolism, such as reductions in juvenile growth rates and spawning, threatening the global and sustainable aquaculture industry of abalone36. To better understand the reproductive mechanism in the Pacific abalone Haliotis discus hannai (Hdh), we recently analyzed the neural ganglia transcriptome and peptidome associated with sex, growing stage, and sexual maturation of this species37,38. Given that NPF/Y signaling is known to regulate food intake in both vertebrates and invertebrates9,10,20, we hypothesized that Hdh-NPF regulates food intake in Pacific abalone. We here report the identification and characterization of a functional Hdh-NPF signaling system. The novel molluscan Hdh-NPFR can provide further insights into the evolution and function of NPF/Y receptors across bilaterian organisms.
Results
Sequence analysis of Hdh-NPF precursors
The lengths of the nucleotide sequences predicted to be Pacific abalone NPF transcripts (Hdh-NPF) were 621 bp and 647 bp, including a 5′-untranslated region (UTR), 3′-UTR, and poly(A) tail (Supplementary Fig. S1). Two prepro-Hdh-NPF transcripts had identical sequences, except for the 26-nucleotides insertion in the transcript with a longer splicing variant. The coding sequences of the prepro-Hdh-NPF transcripts encoded 81 aa, including a 21-aa signal peptide and a 39-aa mature Hdh-NPF peptide. A cleavage site was identified at the end of mature Hdh-NPF with a G residue responsible for biological amidation. Mature NPF/Y peptide sequences from representative species in the phyla Mollusca, Annelida, Platyhelminthes, Arthropoda, Chordata, and Nematoda were compared as shown in Fig. 1a. RPRF in the C-terminal and P8,11 residues, which are known to be important for NPY receptor affinity39, were well conserved among the NPF/Y orthologs. Further mapping of the Hdh-NPF gene revealed that two prepro-Hdh-NPF precursors were transcribed from two different Hdh-NPF genes (Fig. 1b), which are on the same scaffold in a stretch of 45.9 kb40. Approximately 7.2 and 8.7 kb-long Hdh-NPF genes comprised 4 exon–3 intron and 5 exon–4 intron structures, respectively, and shared a relatively high sequence similarity (63.7%). The nucleotide sequence identities between two Hdh-NPF genes of exon 2, 3, and 4 were 91%, 100%, and 96%, respectively. The first and second exons (E1 and E2) of the shorter 7.2 kb-long gene commonly encoded 5′-UTRs, signal sequences, and the majority of Hdh-NPF peptide regions in two prepro-Hdh-NPF precursors, whereas the last two exons (E4 and E4′) of the 8.7 kb-long gene were alternatively spliced for the two transcripts (Fig. 1a,b). The second intron located between the second and the third nucleotide of the second R codon in the C-terminal RxRF/Yamide motif of Hdh-NPF peptide was strictly conserved with the corresponding exon–intron borders in other vertebrate NPY and invertebrate NPF genes (Fig. 1a,c). Using the maximum likelihood method, a phylogenetic analysis was performed with bilaterian NPF/Y, invertebrate deuterostome sNPF/PrRP precursors, and protostome sNPF precursors, along with two molluscan GnRH precursors as outgroups. This showed that the aa sequence of the Hdh-NPF precursor was grouped with those of the molluscan NPF precursors into a lophotrochozoan NPF subfamily (Supplementary Fig. S2).
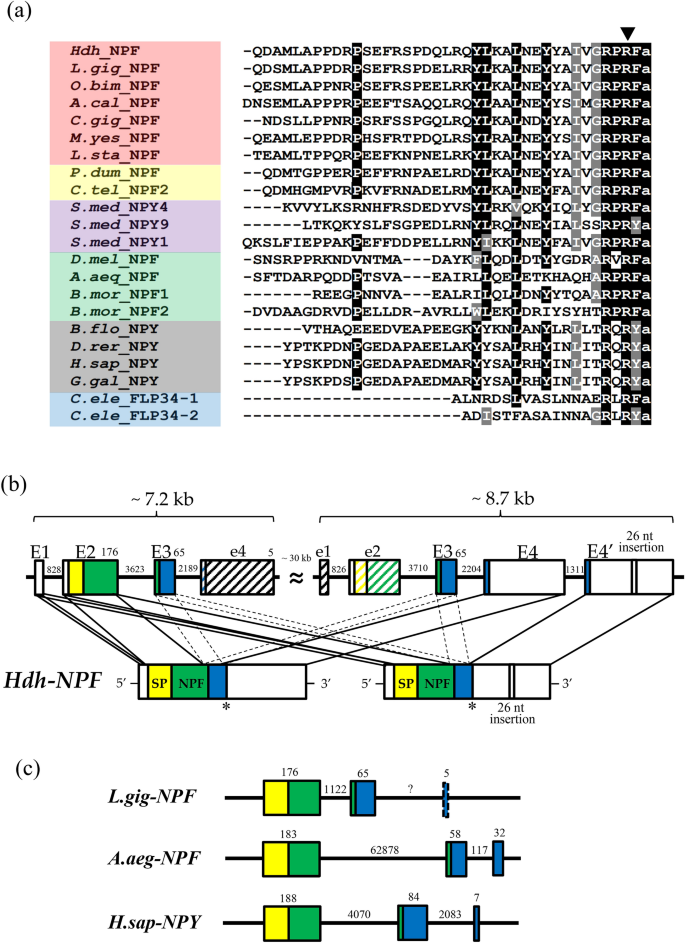
NPF and NPY peptides with H. discus hannai (Hdh) NPF genomic structure. (a) Comparison of the amino acid sequence of Hdh-NPF peptide with those of related NPF/Y peptides from other phyla. Black and grey shaded amino acids represent identical and similar residues, respectively, with a minimum of 70% conservation. Exon–intron boundary is represented by the downward solid arrowhead. The color coding of phyla is as follows: red (Mollusca), yellow (Annelida), violet (Platyhelminthes), green (Arthropoda), grey (Chordata), blue (Nematoda). The full name of the species and the accession numbers of the sequences are listed in Supplementary Table S1. (b) Schematic representation of two Hdh-NPF transcripts with corresponding NPF/NPY genes in the Hdh genome (accession number PRJNA317403) and (c) Lottia gigantea (L.gig; NW_008710370.1), Aedes aegypti (A.aeg; NC_035107.1), and Homo sapiens (H.sap; NG016148) genomes. Signal peptides (SP), the mature NPF/Y, and the NPF/Y-associated peptide are shown by yellow, green, and blue boxes, respectively, with 5′- and 3′-untranslated regions shown in white boxes. Exons (E1–E4′) and introns are indicated by boxes and lines, respectively. Dotted lines linked to two E3 represent undefined joining of E3 between E2 and E4/E4′. Hatched boxes labeled with e1, e2, and e4 indicate highly similar sequences to E1, E2, and E4, respectively, but have different reading frames from Hdh-NPF, because of frameshift mutations. Numbers on boxes and lines indicate the length of nucleotides.
Sequence analysis of Hdh-NPFRs
Four putative abalone NPF receptors (Hdh-NPFRs) were identified by a BLAST search with L. stagnalis NPFR (L.sta_NPFR or L.sta_GPCR105) and human NPYRs. Among them, we named the NPFR with the highest aa sequence identity (57.4%) to L.sta_NPFR as Hdh-NPFR (373 aa) and the others were designated Hdh-NPFR-like-1 (419 aa), -2 (377 aa), and -3 (390 aa) in the order of sequence similarity (27.4–22.9%). The abalone receptors had one N-terminal extracellular domain (ECD), seven trans-membrane domains (TMDs), three extracellular and intracellular loops (ECLs and ICLs), and one C-terminal intracellular domain (ICD). We also determined two potential N-glycosylation sites in the ECD and a characteristic E/DRY/F sequence of rhodopsin-like GPCR in the second ICL of Hdh-NPFR (Supplementary Fig. S3). The two glycosylation consensus sequences (N-X-S/T) in the ECD and the E/DRY/F sequence in the second ICL are highly conserved across invertebrate NPFRs and a sNPF/PrRP-type receptor (A.rub_sNPF/PrRP-R) in the starfish Asterias rubens. Two consensus protein kinase C (PKC) phosphorylation sequences (R/K-X-S/T) were observed in the C-terminal ICD of Hdh-NPFR as a similar feature with the consensus PKC and PKA phosphorylation sites (R-X-S/T or R-R/K-X-S/T) in the C-terminal ICD of A.rub_sNPF/PrRP-R, Drosophila NPFR (D.mel_NPFR), and human NPYR1/2. Comparison of the exon–intron structures and coding sequences (CDS) of Hdh-NPFR and Hdh-NPFR-like genes with those of L.sta_GPCR105, A.rub_sNPF/PrRP-R, and human and chicken NPYR1/2/5 genes showed that Hdh-NPFR and Hdh-NPFR-like genes were more similar to A.rub_sNPF/PrRP-R and vertebrate NPYR2 than to NPYR1 and NPYR5, in terms of an intron insertion in the CDS in NPYR1 and a longer third ICL in the CDS of NPYR5 (Supplementary Figs. S3 and S4).
To investigate relationships of the Hdh-NPFR and Hdh-NPFR-like receptors with other bilaterian NPF/Y and related receptors, we performed a phylogenetic analysis using a sequence database with bilaterian NPF/NPY/sNPF receptors and other closely related receptors (tachykinin-, luqin-/RYamide-, PrRP-, and GPCR83-receptors as outgroups). This analysis revealed that the Hdh receptors were positioned in a large bilaterian NPF/Y receptor superfamily, with a bootstrap support of 71% (Fig. 2). More specifically, Hdh-NPFR was nested in the subclade composed of the deorphanized molluscan L.sta_NPFR, echinoderm sNPF/PrRP-Rs, and vertebrate NPYR2/7 with a relatively lower bootstrap value (45%); Hdh-NPFR-like-1 and Hdh-NPFR-like-2/-3 receptors were positioned in the subclades composed of lophotrochozoan and ecdysozoan NPF/Y receptors, and another lophotrochozoan NPFR-like receptors, respectively. All the Hdh-NPFR-like-1/2/3 showed a sister relationship with the subclade composed of vertebrate NPYR1/4/5/8.
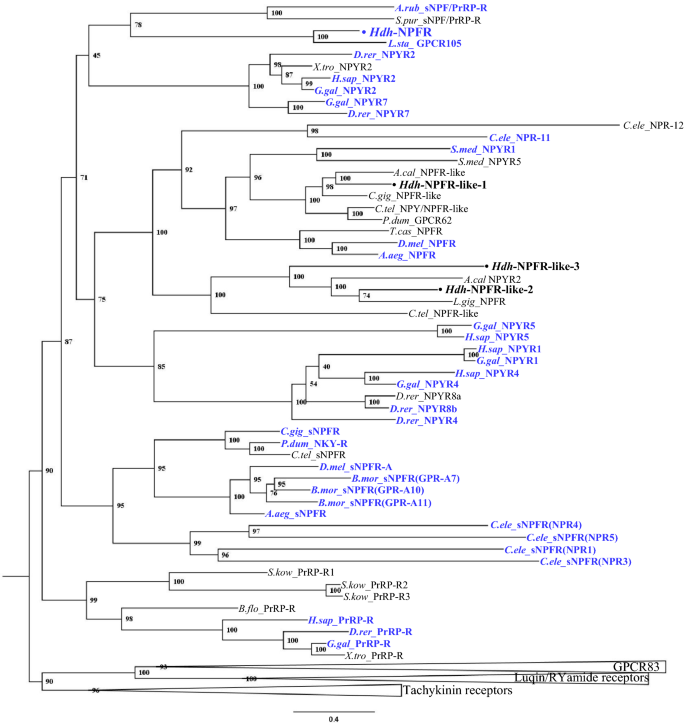
Phylogenetic tree analysis of Hdh-NPFR and three Hdh-NPFR-like receptors with bilaterian NPY/NPF-type, protostome sNPF-type, and deuterostome PrRP-type receptors. Luqin/RYamide-type, tachykinin-type, and GPCR83-type receptors were used as outgroups (condensed). Amino acid sequences of the receptors (Supplementary Table S2) were aligned and trimmed 270 residues were used to generate the maximum likelihood tree using W-IQ server. Ultrafast bootstrap values are given at each branch, and scale bar indicates amino acid substitutions per site. Deorphanized receptors for which receptor-ligand interactions have been experimentally characterized are colored in blue.
Subcellular localization of Hdh-NPFRs
Expression of hemagglutinin (HA)-tagged Hdh-NPFRs in human embryonic kidney 293 (HEK293) cells was verified by immunocytochemistry (ICC) with an anti-HA antibody. ICC analysis revealed that Hdh-NPFR and Hdh-NPFR-like receptors were mainly expressed in the cell membranes, although immunoreactive signals were also detected in the cytoplasm (Supplementary Fig. S5). The potency of Hdh-NPF to induce Hdh-NPFR internalization was determined by ICC. At 30 min post treatment of Hdh-NPF, Hdh-NPFR on the cell membrane moved into the cytoplasm, providing reliable evidence for the interaction of Hdh-NPF with Hdh-NPFR (Supplementary Fig. S6).
Functional characterization of Hdh-NPFRs
To further evaluate the signaling pathways involved in the abalone NPFR-related receptors, luciferase reporter systems under control of a minimal promoter containing a serum response element (SRE-Luc) or cAMP response element (CRE-Luc) were applied to determine ERK/MAPK activity, Ca2+ mobilization, and cAMP accumulation in Hdh-NPFRs-transfected HEK293 cells. Among the four Hdh-NPFR-related receptors, the synthesized Hdh-NPF peptide increased the SRE-Luc activity in the Hdh-NPFR-transfected HEK293 cells in a dose-dependent manner (Fig. 3a,b). Intracellular Ca2+ mobilization profiles of Hdh-NPFR were further investigated in Chinese hamster ovary (CHO)-K1 cells transfected with Hdh-NPFR- and aequorin-expressing plasmids with or without the promiscuous Gα15 plasmid. If Hdh-NPFR activation leads to Ca2+ increase without the promiscuous Gα15, this indicates that the Hdh-NPFR couples to the endogenous Gq protein to activate the phospholipase C (PLC)/inositol trisphosphate/PKC/Ca2+ release pathway in the CHO-K1 cells. Hdh-NPFR-transfected cells generated robust luminescence responses to Hdh-NPF in a dose-dependent manner, regardless of the presence of Gα15 (Fig. 3c and Supplementary Fig. S7a). However, the CRE-Luc-transfected HEK293 cells with or without Hdh-NPFR also responded to an increasing dose of Hdh-NPF, suggesting unknown receptor activity to heterologous Hdh-NPF in HEK293 cells (Fig. 3d). When we analyzed the CRE-Luc reporter system, human NPY inhibited forskolin-stimulated CRE-Luc activities in HEK293 cells expressing NPYR1/2 in a dose-dependent manner (Supplementary Fig. S8), indicating that NPY-mediated NPYR1/2 activation inhibits the cAMP/PKA signaling pathway, consistent with the findings of previous reports39,41.
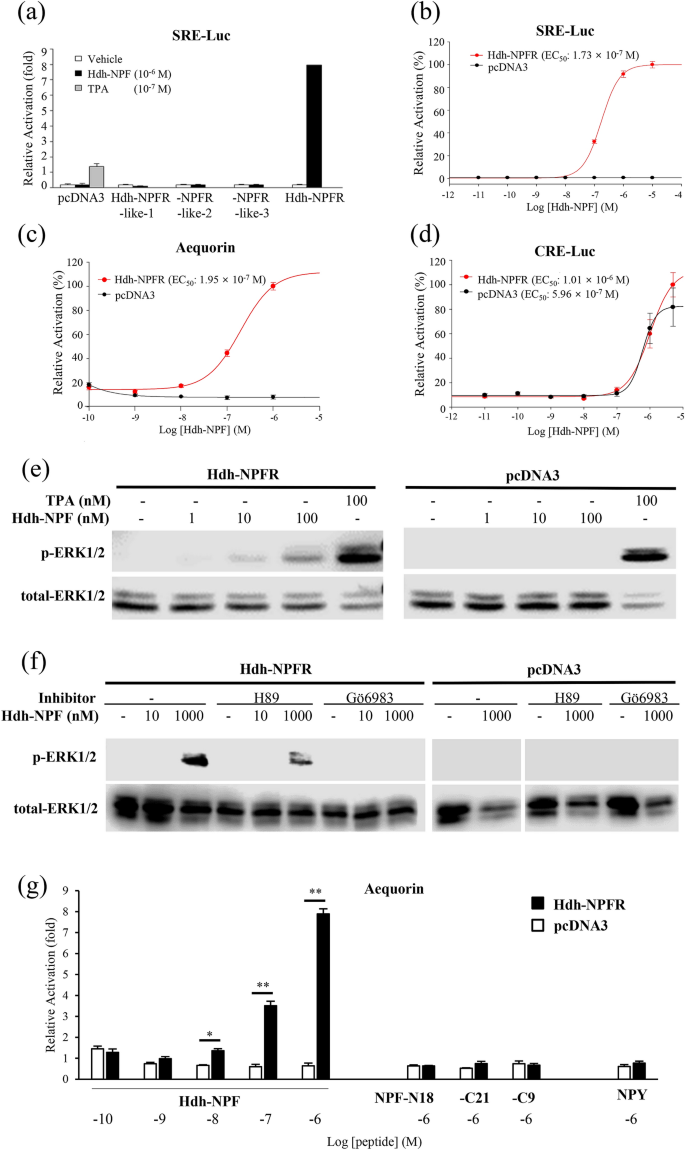
Hdh-NPF peptide induces the Hdh-NPFR signaling pathway. (a) SRE luciferase (SRE-Luc) reporter activities in Hdh-NPFR-related receptor-expressing HEK293 cells treated with Hdh-NPF. (b) Dose–response curve for SRE-Luc activities in Hdh-NPFR-expressing HEK293 cells treated with Hdh-NPF. Dose–response data are shown as percentages relative to the highest value (100% activation). (c) Dose–response curve for intracellular Ca2+ in Hdh-NPFR- and aequorin-expressing CHO-K1 cells treated with Hdh-NPF. (d) CRE-Luc reporter activities in Hdh-NPFR- or pcDNA3-transfected HEK293 cells. (e) Concentration dependence of Hdh-NPF-stimulated phosphorylation of ERK1/2 in Hdh-NPFR-expressing HEK293 cells. (f) Effects of the PKA inhibitor H89 and the PKC inhibitor Gö6983 on Hdh-NPFR-mediated activation of ERK1/2 in HEK293 cells. The cells were pre-incubated with or without the inhibitors (10–5 M) for 1 h and then stimulated with NPF. Full-length blots for phosphorylated and total ERK1/2 are presented in Supplementary Fig. S9. (g) Mean intracellular Ca2+ responses of Hdh-NPFR-transfected CHO-K1 cells by NPF, truncated Hdh-NPF-N18, -C21, -C9, and human NPY (see Table 1). Values for luciferase reporter and Ca2+ response assays are presented as the mean ± SEM (n = 3 or 4). Statistical significance was tested by Student's t-test. *P < 0.05; **P < 0.01. TPA, 12-O-tetradecanoylphorbol-13-acetate; EC50, half-maximal effective concentration; H89, N-[2-(p-bromocinnamylamino)ethyl]-5-isoquinolinesulfonamide.
Hdh-NPF treatments increased ERK phosphorylation in the Hdh-NPFR-transfected cells in a ligand-dependent manner (Fig. 3e). The Hdh-NPFR-mediated activation of ERK1/2 was abolished by the PKC inhibitor Gӧ6983 but not by the PKA inhibitor H89 (Fig. 3f), suggesting a fundamental importance for the Gq-mediated signaling pathway in the ERK1/2 MAPK cascade. In contrast, truncated Hdh-NPF peptides (NPF-N18, -C21, and -C9) and human NPY could not induce the Ca2+ mobilization response in the Hdh-NPFR-transfected cells (Fig. 3g). Two chimeric mixed peptides for Hdh-NPF and human NPY (NPF-Y and NPY-F) could not activate Ca2+ mobilization in the Hdh-NPFR-transfected cells (Table 1; Supplementary Fig. S7b).
In silico model of the NPF-NPFR complex
Docking simulation was performed to predict the binding mode of Hdh-NPF peptide and estimate the critical interactions in the Hdh-NPFR binding pocket. The docking model suggested that the C-terminal sequence of Hdh-NPF peptide mainly interacts with Hdh-NPFR (Fig. 4). Specifically, R38 in abalone NPF forms a salt bridge with E204 and E284 in Hdh-NPFR, maintaining a stable hydrogen bond network. In addition, the I33, V34, and F39 residues in NPF have extensive hydrophobic interactions with Y108, I190, Y201, and V203 in Hdh-NPFR. Due to the small size of the binding pocket, only the C-terminal loop region penetrates the receptor and the rest of the peptide structure is exposed to the solvent. The internal peptide sequence near the helix region of Hdh-NPF (Q22–Y31) has many contact points to ECL3 in Hdh-NPFR (S288–K293), connecting TMD6 and TMD7 outside the binding pocket, which differs from the model of human NPY binding to Hdh-NPFR (Supplementary Fig. S10). This model suggests that the interaction between the specific residues of ECLs and Hdh-NPF peptide is critical for Hdh-NPFR activation.
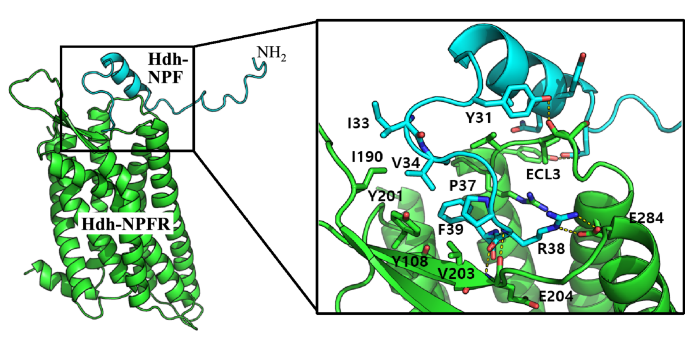
In silico docking model of the NPF-NPFR complex. The cartoon representation for Hdh-NPFR and Hdh-NPF is shown in green and cyan, respectively. The peptide binding interface (black box) is enlarged to show the details of the residue interactions, including R38 of Hdh-NPF and E284 in Hdh-NPFR. The helix region of Hdh-NPF (Q22–Y31) contacts the ECL3 region in Hdh-NPFR (S288–K293) connecting TMD6 and TMD7 outside the binding pocket.
Tissue distribution of Hdh-NPF precursor and Hdh-NPFR transcripts
The expression patterns of prepro-Hdh-NPF and Hdh-NPFR transcripts were investigated in the cerebral ganglia (CG), pleuro-pedal ganglia (PPG), ovary, gills, intestine, and hepatopancreas of female abalone. The prepro-Hdh-NPF transcript was dominantly expressed in the CG, with a significantly higher level found in sexually mature females than in immature females (P < 0.05; Supplementary Fig. S11a). Hdh-NPFR transcripts were mainly expressed in the CG and PPG compared with the other tissues examined (Supplementary Fig. S11b). There were no significant differences of Hdh-NPFR transcript levels between immature and mature abalone tissues.
Effects of Hdh-NPF on food consumption
Since NPF is known to be involved in energy metabolism in diverse invertebrate phyla20, we investigated whether Hdh-NPF affects food intake in Pacific abalone. Abalone injected with Hdh-NPF [2.5 μg/g body weight (BW)] showed a significant increase in food consumption (P < 0.05; Fig. 5a) at 24 h post-injection, whereas food consumption was significantly inhibited by injection of a double-stranded (ds) RNA for Hdh-NPF transcript (dsRNA-Hdh-NPF) compared with that observed following saline injection (P < 0.05; Fig. 5b). A significant decrease in Hdh-NPF transcript levels was also found in the dsRNA-Hdh-NPF-injected abalone group (P < 0.05; Fig. 5c), whereas no significant changes in Hdh-GnRH and Hdh-APGWa transcript levels were detected between saline- and dsRNA-Hdh-NPF-injected abalone groups (Supplementary Fig. S12).
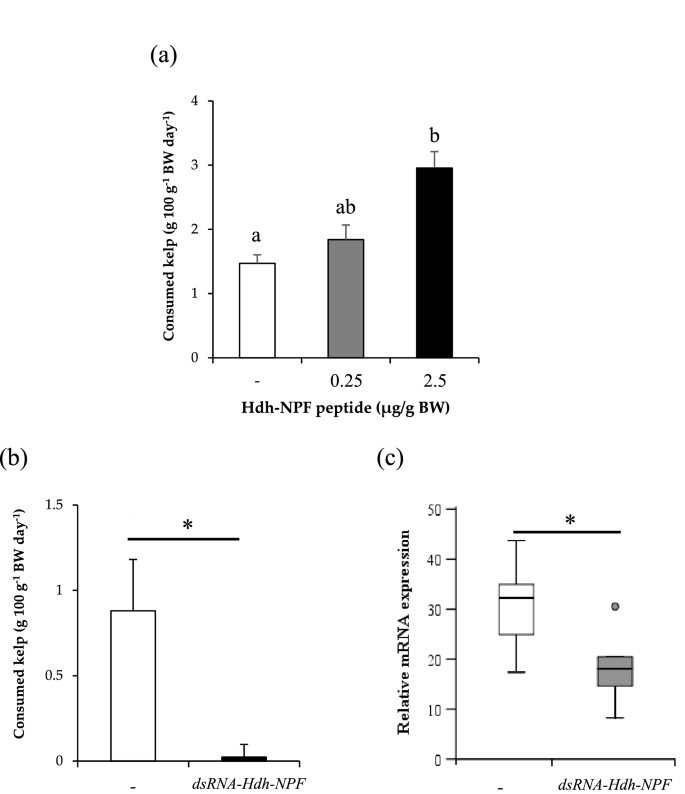
Effect of Hdh-NPF and double-stranded RNA of Hdh-NPF mRNA on food intake in abalone. (a) Effect of in vivo administration of Hdh-NPF peptide on kelp consumption in abalone. Each column and bar represent the mean and SEM (n = 11 per group), respectively. Significances of differences were evaluated by one-way analysis of variance with the Bonferroni correction method in comparison with the mollusk saline-injected group. Different lowercase letters indicate significant differences between treatments (P < 0.05). (b) Effects of Hdh-NPF knockdown on food intake. For RNA interference, individual abalone were injected with 165 μg of dsRNA-Hdh-NPF or the same volume of mollusk saline. Feeding assays were performed on the third day after injection. Results represent means ± SEM (n = 10 per group); *significant difference (P < 0.05) by Student's t-test. (c) Relative Hdh-NPF transcript levels in the cerebral ganglia of abalone. The Hdh-NPF transcript levels in dsRNA-Hdh-NPF- or saline-injected abalone were measured by RT-qPCR. The ribosomal protein L-5 (Hdh-RPL5) was used as the internal control. Results represent means ± SEM (n = 10 per group); *statistical significance (P < 0.05) by Mann–Whitney U test.
Discussion
In-depth screening of databases of diverse bilaterian genome and transcriptome sequences strongly suggested an ancient co-evolutionary history between NP precursor and receptor genes42,43. Elucidation of the endogenous ligands for orphan receptors in D. melanogaster and C. elegans has also offered important insight into the origin of NP signaling systems, dating back to the emergence of protostomes44,45. However, the NP systems of model species do not fully represent neofunctionalization and/or subfunctionalization of the ligand-receptor families of diverse animal phyla. Here, we present evidence that the abalone Hdh-NPF and Hdh-NPFR are evolutionally and functionally orthologous to the vertebrate NPY signaling system.
We identified two transcripts encoding a common Hdh-NPF precursor including the identical Hdh-NPF peptide sequence. Although a difference in a 26-nucleotides sequence at 3′-UTRs was found in the two Hdh-NPF transcripts, they were found to commonly encode a complete and identical open reading frame of Hdh-NPF precursor. The tandem Hdh-NPF genes were on the same scaffold in Hdh genome40, as seen in several invertebrate NPF/Y genes4,46. Given the conserved exon–intron structure and sequence similarities, it is likely that the two Hdh-NPF genes evolved from an initial gene duplication event at the chromosome level in Pacific abalone. A majority of NPF peptides have the evolutionally conserved C-terminal RxRF/Yamide sequences in diverse protostome invertebrates19,20,21. The molluscan NPF peptides including Hdh-NPF peptide comprised the conserved C-terminal RxRF/Y-amide and N-terminal PxxP residues, which are important for receptor affinity in vertebrate NPY signaling system39. In contrast, a phylogenetic analysis could not show a clear relationship between NPF/Y-related subfamily members, although the Hdh-NPF precursor was positioned in a clade of molluscan NPF group with a relatively high bootstrap value. This may be because of the variable signal peptide and the extensive divergence of C-terminal extension, resulting in the aa sequences to be of little or no use for large evolutionary distances. However, we determined that the exon–intron borders, especially the interposition of introns between the second and the third nucleotide of the second R codon in the C-terminal RxRF/Yamide motif, were highly conserved in the bilaterian NPF/Y genes and FLP-34 gene, a NPF/Y ortholog in C. elegans21. These findings strongly suggest that the Hdh-NPF precursor is a genuine member of the invertebrate NPF group and that the bilaterian NPF/NPY genes originated from a common ancestor gene.
To date, L.sta_NPFR has been the only functionally verified receptor for molluscan NPF20,30. In this study, we identified the second molluscan NPFR from Pacific abalone that is characterized as a rhodopsin-like GPCR, with three ICDs and three ECD loops, an E/DRY/F sequence at the beginning region in the second IL, and seven TMDs47,48. The E/DRY/F sequence was highly conserved among the compared NPF/Y receptors, suggesting that the E/DRY/F motif plays a critical role in receptor function. For instance, mutation of the Arg residue in the E/DRY/F motif of human GPR40 (free fatty acid receptor 1), resulted in loss of agonist-induced functions, including Ca2+ mobilization, ERK activation, and receptor internalization49. PKC phosphorylation sites in the C-terminal ICD were similarly positioned in the Hdh-NPFR, D.mel_NPFR, A.rub_sNPF/PrRP-R, and human NPYR1/2, whereas consensus glycosylation sites were found within the predicted extracellular N-terminus of all the examined receptors. This suggests that the potential PKC phosphorylation sites in the ICD are not likely involved in the pivotal function of NPF/Y receptors, although the PKC-dependent cascade is clearly involved in activation of Hdh-NPFR as described in further detail below.
Through the sequence comparison and phylogenetic analysis, here we report that Hdh-NPFR-related receptors are belong to the NPF/Y receptor family distinct from the sNPFR and PrRP-R families in bilaterians. Consistent with this analysis, recent phylogenetic studies with novel NPF/NPY/PrRP/sNPF-related receptors in echinoderms and three lophotrochozoans (nemerteans, brachiopods, and phoronids) have revealed that all the examined molluscan NPFR-like sequences are orthologous to the vertebrate NPY receptors22,43. In addition, we suggest that Hdh-NPFR is most likely similar to vertebrate NPYR2 among the three vertebrate NPYR ancestors, the progenitors of the NPYR1, NPYR2, and NPYR5 subfamilies4,50. The first line of evidence is the sequence similarity between NPYR2 and Hdh-NPFR based on the BLAST and a phylogenetic analysis. Hdh-NPFR showed connections with the vertebrate NPYR2/7 cluster than with the NPYR1/4/5/8 cluster, although the bootstrap value of the branch was relatively low in this phylogenetic tree. In a previous report, the sequences of deorphanized L.sta_NPFR and D.mel_NPFR were closely related to the vertebrates NPYR2 receptor subtype in a phylogenetic tree based on Clustal W alignment and Jukes-Cantor distance analysis51. The second line of evidence is the exon–intron structure and the aa length of the third ICL of NPF/Y receptors. In human, chicken, and coelacanth fish (Latimeria chalumnae), a representative of a basal lineage of vertebrates, NPYR1 show a conserved intron interposition in the nucleotide sequence encoding the 5th TMD region, whereas NPYR2 and NPYR5 have no intron sequences in the corresponding regions52,53,54,55,56. Similarly, Hdh-NPFR and Hdh-NPFR-like receptors had no interposition of introns in the entire open reading frame region. The third ICLs of vertebrate NPYR5 is at least four times longer than the corresponding region of other NPYR subtypes, which is clearly distinguishable from Hdh-NPFR and Hdh-NPFR-like receptors. Interestingly, comparison of the sequence of Hdh-NPFR with the starfish A.rub_sNPF/PrRP-R showed several common features, including the aforementioned predicted E/DRY/F motif in the second ICL, PKC phosphorylation sites in the C-terminal ICD and the exon–intron structure. However, functional studies on Hdh-NPFR and Hdh-NPFR-like receptors are needed for further characterization of molluscan NPFR gene duplication and the ancient complexity of the vertebrate NPY signaling system, since the L.sta_NPFR and A.rub_sNPF/PrRP-R were assumed to be vertebrate NPYR1 and PrRP-R homologs, respectively, based on pharmacological data22,33.
Since an earlier pioneering study demonstrated that Ls-NPF can activate the cognate receptor L.sta_NPFR in a model molluscan species, L. stagnalis30, the characterization of other functional NPF receptors and their downstream signaling pathways in mollusks has long been awaited. Here, we show that Hdh-NPFR is potently activated by the mature Hdh-NPF, and the ligand-activated receptor leads to activation of MAPK/ERK signaling through a Gq-PLC-PKC-dependent cascade and increase of intracellular Ca2+ mobilization, as is the case for L.sta_NPFR, human NPYR2, and chicken NPYR1/2/5/7 receptors13,30,54,55. This provisional pathway is supported by the results of western blot analyses showing that the PKA-specific inhibitor failed to inhibit the phosphorylation of ERK1/2, whereas the PKC inhibitor inhibited ERK1/2 phosphorylation in Hdh-NPFR-expressing cells after Hdh-NPF treatment. Nevertheless, we cannot rule out the possible inhibitory pathway of cAMP/PKA in the liganded Hdh-NPFR signal transduction system. In fact, L.sta_NPFR and vertebrate NPYRs are functionally coupled to more than one secondary messenger system13,30,54,55. The Hdh-NPFR along the cellular membrane translocated to an intracellular compartment in HEK293 cells at 30 min post treatment of Hdh-NPF, similar to NPY-stimulated human NPYR157, suggesting that Hdh-NPFR transduces Ca2+ responses rapidly through the Gq-PLC-PKC signal cascade. Among the three vertebrate NPYR ancestors, NPYR1, NPYR2, and NPYR5, the most prominent pharmacological feature of NPYR2 is that it can bind N-terminal truncated peptide fragments12,50. In contrast, truncated Hdh-NPF peptides could not increase the intracellular Ca2+ mobilization in Hdh-NPFR-expressing cells, similar to the response of L.sta_NPFR-expressing cells treated with N-terminal truncated Ls-NPF30. These observations imply that the molluscan NPF signaling systems are not similar to those of vertebrate NPYR2, adding another level of complexity presented by the variation of bilateian NPF/Y system.
To identify the receptor residues that are important for Hdh-NPF binding, we performed a docking simulation to predict the binding mode of Hdh-NPF peptide and estimated the critical interactions in the Hdh-NPFR binding pocket. The docking model suggested that the C-terminal I33–F39 residues of Hdh-NPF are the major sites interacting with the binding pocket of Hdh-NPFR, under the reasonable assumption that R38 of Hdh-NPF interacts with E284 of TMD6 in the Hdh-NPFR extracellular region as the salt bridge formation between R35 and D287 in human NPYR139,58. These data suggest that the ionic interaction contributed by the acidic E/D residue in TMD6 is critical for recognition of the ligands with a positive charge across bilaterian NPF/NPY receptors, including human NPYR259. Similarly, the R33 residue in human NPY preferentially interacts with D292 (corresponding to E284 and D287 in Hdh-NPFR and NPYR1, respectively) in human NPYR239,60. In this context, Hdh-NPFR would be a key and ancestral receptor for understanding the evolution of the NPY signaling system. In addition, we observed that the helix region of Hdh-NPF (Q22–Y31) contacts the ECL3 region of Hdh-NPFR (S288–K293) connecting TMD6 and TMD7 outside the binding pocket, which can explain the stronger energy of Hdh-NPF binding than that of human NPY binding to Hdh-NPFR. Taken together, the docking model presented plausible binding mode of Hdh-NPF with the conserved key interactions.
Since Stanley and Leibowitz61 revealed that injection of NPY into the hypothalamic paraventricular nucleus stimulates food intake in rats, the role of NPY/F signaling in regulation of feeding has been widely demonstrated in diverse vertebrate and invertebrate phyla20. In this study, a high dose of Hdh-NPF peptide injection could increase seaweed consumption, whereas injection of dsRNA for Hdh-NPF mRNA remarkably decreased food intake in Pacific abalone. These findings suggest that NPF production induces feeding behavior with a consequent increase of food intake, as observed across bilaterian species. In fact, feeding rhythms, ingestion rate, and digestive enzyme activities were positively correlated with the gene expression levels of Hdh-NPF in Pacific abalone62 and injection of NPF peptide was reported to significantly increase the filter feeding rate in the molluscan bivalve R. philippinarum31. In contrast, Ls-NPF showed no short-term effect on food intake in L. stagnalis33,34 and its role seems to be quite different from those of abalone NPF and mammalian NPY9,10. Instead, a significant increase in food intake started at 9 days after implantation of Ls-NPF34, suggesting that NPF is a long-term effector of food consumption in L. stagnalis. The possible roles of NPF in these regulatory mechanisms underlying food intake should be further determined in diverse molluscan species.
In conclusion, Hdh-NPF, Hdh-NPFR, and Hdh-NPFR-like receptors are eligible molecules to extend our understanding of the evolution and diversity of the metazoan NPF/Y-mediated signaling system. This work can contribute relevant information for practical applied in monitoring the physiological homeostasis and metabolism of Pacific abalone as an important economic species that is under threat due to extensive environmental stress from farming to meet the high market demand. Further elucidation of NPs with their cognate receptors in mollusks can provide opportunities to search endogenous NPs for uncharacterized human GPCRs, and to develop pharmaco-therapeutic ligands for human NP signaling systems.
Materials and methods
Nucleotide/amino acid sequence and phylogenetic analyses
Transcripts for the Hdh-NPF precursors (NCBI GenBank accession numbers MZ027150–MZ027151), Hdh-NPFR (MZ014382), and Hdh-NPFR-like receptor sequences (MZ014383–MZ014385) of Pacific abalone, H. discus hannai, were previously determined and were obtained from transcriptome databases37,38. Functional annotations were conducted by comparing the sequences against those in public databases, including the National Center for Biotechnology Information (NCBI) BLASTp program. Sequence alignments for bilaterian representative NPF/Y peptides (Supplementary Table S1) were performed using Clustal Omega Multiple Sequence Alignment with default parameters63. The software BOXSHADE (https://embnet.vital-it.ch/software/BOX_form.html) was used to highlight conserved amino acids. Sequence alignments for Hdh-NPFR-related receptor sequences were performed using CLC Genomics Workbench software. The prediction of transmembrane helices in Hdh-NPFR-related receptors was performed using the latest version of the TMHMM program64. The N-linked glycosylation and intracellular phosphorylation sites were predicted by the NetNGlyc and NetPhos servers, respectively (https://services.healthtech.dtu.dk/). The exon–intron boundaries were predicted by BLAST of NCBI Genome Workbench.
To generate phylogenetic trees, aa sequences of bilaterian NPF/Y and sNPF prepro-hormones (Supplementary Table S1), and NPF/Y, sNPF, and PrRP receptors (Supplementary Table S2) were retrieved from references21,22 and the NCBI nr repository. In total, 30 prepro-hormones and 83 receptors were aligned using MUSCLE in the online tool NGPhylogeny (iterative, 16 iterations, UPGMB as clustering method)65 and automatically trimmed using trimAL in the online tool NGPhylogeny66. The trimming contained a total of 39 and 270 residues for prepro-hormones and receptors, respectively, that were used to generate the maximum likelihood tree using W-IQ server v1.6.1267. The substitution models, PMB + I + G4 for prepro-hormones and LG + F + I + G4 for receptors, and the ultrafast bootstrap approximation approach and SH-aLRT 1000 replicates were used. Phylogenetic trees were visualized, using the free software package FigTree v1.4.3 by A. Rambaut at http://tree.bio.ed.ac.uk/software/figtree/.
cDNA cloning and plasmid construction
Female Pacific abalone (9.2 cm shell length; 91.2 g BW) was purchased from a local dealer (Gangneung, Gangwon-do, Korea). To...
Comments
Post a Comment